1.1 Land Management Plan vol 1 draft — original pdf
Backup
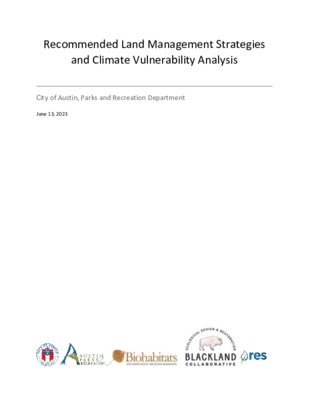
Recommended Land Management Strategies and Climate Vulnerability Analysis City of Austin, Parks and Recreation Department June 13, 2023 Acknowledgements This Climate Vulnerability and Land Management effort would not have been possible without the generous participation of numerous individuals. We would like to thank everyone who volunteered their time to participate in numerous conversations, meetings, and field work so that we could shape a plan that reflects the values of Austin. The Lady Bird Johnson Wildflower Center was particularly helpful in field assessments. We also want to thank everyone who took time out of their busy schedules to review the draft language of this report and provide comments. Each comment was thoroughly reviewed, and your recommendations helped shape this plan. COA Staff in Austin Parks and Recreation with valuable contributions from Austin Fire Department Wildfire Division, Austin Public Health, Austin Water - Balcones Canyonlands Preserve, City of Austin Equity Office, Office of Sustainability, Office of Resilience, and Watershed Protection Department. Consulting Team Blackland Collaborative Inc. Biohabitats Inc. RES Inc. 1 Introduction and Background ............................................................................................................... 1 Contents 1.1 1.2 Statement of Need Overview of Approach 1.2.1 Plan Scope ............................................................................................................................. 1 1.2.2 Plan scope ............................................................................................................................. 2 1.3 Relation to Other Plans and Studies 1.3.1 Climate, Park, and Sustainability Plans ................................................................................. 6 1.3.2 Land Management Plans ....................................................................................................... 7 2 Vision and Goals.................................................................................................................................... 9 2.1 2.2 2.3 3.1 3.2 3.3 3.4 Land Acknowledgement Mission Goals Investment Gaps and Equity Mapping Natural Areas and Human Health 3 Social Equity ........................................................................................................................................ 12 3.1.1 Physical health .................................................................................................................... 12 3.1.2 Mental health ...................................................................................................................... 12 3.1.3 Economics ........................................................................................................................... 13 3.1.4 Safety .................................................................................................................................. 14 Land Conservation in Austin Engagement and Decision Making 4.1 Regional Setting 4 Ecological Management Framework .................................................................................................. 20 4.1.1 Ecoregions ........................................................................................................................... 20 4.1.2 Geology ............................................................................................................................... 29 4.1.3 Hydrology ............................................................................................................................ 30 4.1.4 Soils ..................................................................................................................................... 33 4.1.5 Plant Community Types ...................................................................................................... 35 4.1.6 Habitat................................................................................................................................. 36 4.1.7 Land Use .............................................................................................................................. 37 4.2 Ecological Site Descriptions i 1 1 6 9 9 11 12 12 14 18 20 37 4.2.1 State and Transition Pathways ............................................................................................ 37 4.2.2 Ecological Site Summaries ................................................................................................... 39 5 Climate Vulnerability Analysis ............................................................................................................ 42 5.1 Climate Change Summary 5.1.1 CO2 concentrations and emissions scenarios ..................................................................... 42 5.1.2 Temperature ....................................................................................................................... 42 5.1.3 Precipitation ........................................................................................................................ 43 5.1.4 Evaporative demand ........................................................................................................... 44 5.1.5 Austin’s Timeline for Change .............................................................................................. 46 5.2 Ecosystem Components 5.3 Climate Analogues 5.2.1 Abiotic Components ............................................................................................................ 47 5.2.2 Biotic Factors ....................................................................................................................... 48 5.3.1 COA-generated analogues ..................................................... Error! Bookmark not defined. 5.3.2 Interpreting Climate Analogues .......................................................................................... 56 5.4 Climate-driven Natural Area Outcomes 5.4.1 Canopy Mortality ................................................................................................................ 57 5.4.2 Community Composition .................................................................................................... 57 5.4.3 Reduced Recruitment ......................................................................................................... 57 5.4.4 Intense Wildfire ................................................................................................................... 57 5.4.5 Novel Flood Dynamics ......................................................................................................... 58 Summary of Climate Vulnerability Literature 5.5 5.6 5.7 Climate Smart Strategies Geospatial Analysis 5.7.1 Preliminary System-wide Climate Hazards ......................................................................... 60 5.7.2 Condition Assessment Inputs .............................................................................................. 71 5.7.3 Park-level Climate Vulnerability Assessment Method ........................................................ 76 5.7.4 Geospatial Vulnerability Assessment Results ..................................................................... 77 6 Land Management .............................................................................................................................. 85 6.1 OVERALL MANAGEMENT APPROACH 6.1.1 TARGET COMMUNITIES ...................................................................................................... 85 6.1.2 FUEL TYPES .......................................................................................................................... 87 6.1.3 FIRE RESILEINT LANDSCAPES .............................................................................................. 87 42 47 52 56 58 59 60 85 ii 6.2 ECOSYSTEM SERVICES AND ECOSYSTEM FUNCTION 6.2.1 ECOSYSTEM SERVICES ........................................................................................................ 89 6.2.2 ECOSYSTEM FUNCTION ...................................................................................................... 89 6.3 MANAGEMENT ACTIONS BY COMMUNITY TYPE 6.3.1 WOODLAND MANAGEMENT ............................................................................................. 94 6.3.2 GRASSLAND MANAGEMENT ............................................................................................ 104 6.3.3 BOTTOMLAND/RIPARIAN MANAGEMENT ...................................................................... 108 6.3.4 RARE AND ENDANGERED SPECIES ................................................................................... 111 7 Monitoring & Adaptive Management .............................................................................................. 115 6.4 PRIORITIZING IMPLEMENTATION 7.1 Monitoring Parameters 7.1.1 Potential Fire Behavior ...................................................................................................... 115 7.1.2 Vulnerability Indices .......................................................................................................... 115 7.1.3 Biodiversity and habitat quality ........................................................................................ 116 7.1.4 Habitat Connectivity ......................................................................................................... 116 7.1.5 hydrologic function ........................................................................................................... 116 89 94 113 115 117 118 8 References ........................................................................................................................................ 119 7.2 7.3 Adaptive Management Next steps/policy intersections iii List of Figures Figure 1-1. Austin’s PARD lands in the study area. Balcones Canyonlands Preserve (BCP) land management plans and the Balcones Canyonlands Conservation Land Permit governing documents supersedes this plan on affected lands. ....................................................................... 4 Figure 3-1. City of Austin’s conserved lands (Balcones Canyonlands Preserve, Water Quality Protection Lands, and parks) and 2020 Social Vulnerability Index (SVI) for Travis County. SVI data from 2020, sourced from the US Dept of Health and Human Services. ................................................. 17 Figure 4-1. Ecoregions in the study area. Source: U.S. Level III and IV Ecoregions (U.S. EPA). .................. 21 Figure 4-2. Geology of the study area. Source: State Geologic Map Compilation (SGMC) geodatabase of the conterminous United States (2017) ........................................................................................ 29 Figure 4-3. Watersheds of the study area. Watershed boundaries delineate subbasins that drain to a portion of a stream network. Hydrologic unit codes (HUCs) designations 8 and 12 refer to regional and local scales, respectively. Source: USGS National Hydrography Dataset (NHD). ..... 31 Figure 4-4. Major aquifers in the study area. The Edwards and Trinity Aquifers -- two of Texas’ nine major aquifers - are found in the study area. Aquifer outcrops, or recharge zones, are present in the west where the formations are exposed at the surface, whereas the aquifers are buried in the subcrop areas in the east. Source: TWDB, December 2006. ................................................... 32 Figure 4-5. Soils of PARD lands. Natural Resource Conservation Service (NRCS) identifies five dominant soil orders in the park lands -- mollisols, vertisols, inceptisols, entisols and alfisols in order of abundance -- which refer to their different formations and characteristics. ................................ 34 Figure 4-6. Dominant plant community types in PARD lands. Community types derived from site assessment performed as part of this project. .............................................................................. 35 Figure 4-7. Habitat areas in the study area. National datasets identify areas of recognized biodiversity, general habitat cores (ie not species specific), and connectivity importance for conservation planning. ........................................................................................................................................ 36 Figure 5-1. Vapor pressure diagram. The red area indicates the temperature range in which we are operating with respect to climate change by 2100 (mean summer high temperatures increasing from 34C pre-2011 to almost 40C post-2070). In this range, the change in VPD as a function of temperature is steep. .................................................................................................................... 45 Figure 5-2. Vapor Pressure compared to temperature. As temperature increases, RH decreases (assuming dew point remains constant). Thus, VPD increases slightly exponentially. Orange arrows are for the low emissions scenario. Red arrows are for high emissions scenario. Temperature projections are taken from Hayhoe (2014). Average RH is from summer 2017. Under high emissions scenarios, VPD increases ~60% by 2100. ................................................... 46 Figure 5-3 Post-drought tree mortality. Comparison of four Texas species from Johnson et al. 2018 ...... 50 Figure 5-4. Climate similarity maps Climate similarity maps for Austin in 2080 under unmitigated (RCP8.5) and more optimistic (RCP4.5) emissions scenarios, respectively. Lines (red in A, blue in B) connect Austin to the city (Nuevo Laredo, Mexico) with the highest similarity to Austin’s projected 2080 climate in each scenario. https://fitzlab.shinyapps.io/cityapp/. ......................... 52 Figure 5-5a and 5-5b. Beck et al. (2018) project that Austin’s Köppen-Geiger climate classification (Cfa, i.e., temperate, no dry season, hot summer) under RCP8.5 (unmitigated emissions) will remain unchanged. Its proximity to the adjacent BSh (hot arid steppe) system, however will increase as the BSh zone expands to the north and east. ................................................................................ 54 iv Figure 5-7. COA-created temperature and rainfall analogues Locations in North America where Austin’s projected temperature and rainfall currently occur (based on Jiang and Yang, 2012). ................ 55 Figure 5-8. Crown fire probability in PARD lands within study area. .......................................................... 62 Figure 5-9. Crown fire probability at Trevino Metro Park. ......................................................................... 63 Figure 5-10. Extreme heat in PARD lands within study area. ..................................................................... 65 Figure 5-11. Soil water availability in PARD lands within study area. ........................................................ 66 Figure 5-12. Soil water availability in Trevino Metro Park. ......................................................................... 67 Figure 5-13 Flood mapping for PARD lands within study area. .................................................................. 69 Figure 5-14. Plant community condition for management areas based on 2022 rapid assessments. ....... 72 Figure 5-15. Environmental Vulnerability Index for PARD lands within study area. The index shows the combined risk layers for crown fire, heat and soil water availability . .......................................... 77 Figure 5-16. Environmental Vulnerability Index for Trevino Metro Park as an example of site scale mapping. ........................................................................................................................................ 78 Figure 5-17. Social Vulnerability Index for PARD lands within study area. The index shows the combined variables that identify the differing levels of potential vulnerability at the census tract level. .... 80 Figure 5-18. Social Vulnerability Index for Trevino Metro Park as an example of site-scale summary mapping. ........................................................................................................................................ 81 Figure 5-19. Park Climate Vulnerability Index combining environmental and social vulnerability in the study area. ..................................................................................................................................... 82 Figure 5-20. Park Climate Vulnerability Index combining environmental and social vulnerability as an example of site scale mapping. ...................................................................................................... 83 Figure 5-21. Summary Ranking of Park Vulnerability Index for PARD lands in study area. ........................ 84 Figure 6-1. Ecosystem function. Stepwise degradation of hypothetical natural community illustrating two common transition thresholds that separate the three vegetative groups emphasized here. Their functional integrity and transition limitations rather than species composition define these groups. Natural communities controlled by biotic interactions require some form of vegetation manipulation before recovery can occur. Transition thresholds controlled by abiotic limitations require physical manipulations that increase infiltration, reduce erosion, capture organic materials and/or ameliorate microenvironmental extremes. Adapted from Whisenant 2002. ... 90 Figure 6-2. Organic carbon density above ground (green bars) and below ground (brown bars) is dependent on ecosystem. Adapted from (Amthor et al. 1998). ................................................... 92 Figure 6-3. Native Prairie mix green roof in Wimberley Texas. The roof is growing in shallow media (6 inches) giving the community no access to water stored deeper in the soil column. Its position on a roof top exposes it to more extreme fluctuations in temperature than those on the ground (Simmons 2015). The image on the left shows the roof after being deprived of water for three months (April-June). The image on the right shows the community’s recovery following the application of water. .................................................................................................................... 106 v List of Figures Table 1 Major pre-European savanna and woody forest community types with Ashe juniper as an important component in the Central Texas Hill Country (Excerpted from Diamond 1995). .......................................... 27 Table 2. Abiotic site types were summarized based on analysis of 30-m resolution digital elevation models and then overlaid the 30-m resolution satellite-derived land cover such as the national land cover database. Adapted from Diamond and True 2008. ....................................................................................................... 28 Table 3. Assuming no change in average summer dew point temperature (the authors’ assumption), by 2040, the average daily summer high vapor pressure deficit is projected to rise by 17.1%. By 2100, average daily summer high VPD is projected to rise by 27% and 60.1% under the low and high emission scenarios. Temperature anomalies are taken from (Hayhoe 2014). Average summer low RH is from 2017 weather data. .............................................................................................................................................................. 45 Table 4. Hazard indicators ........................................................................................................................................... 70 Table 5. Soil Condition Classes..................................................................................................................................... 73 Table 6 Community Condition Classes......................................................................................................................... 86 Table 7 Woodland Goals and Strategies .................................................................................................................... 100 Table 8. Grassland Goals and Strategy Summary ...................................................................................................... 107 Table 9 Bottomland Goals and Strategy .................................................................................................................... 110 Select Acronyms Balcones Canyonland Preserve City of Austin Austin/Travis County Community Wildfire Protection Plan Environmental Protection Agency Federal Emergency Management Agency Geographic Information System Natural Resources Conservation Service Texas Parks and Wildlife TWDB Texas Water Development Board USGS U.S. Geological Survey USFWS U.S. Fish and Wildlife Service BCP COA CWPP EPA FEMA GIS NRCS TPW vi Executive Summary Functional ecosystems are central to sustainable cities and human well-being. Public natural areas are vital for human health and well-being, supporting human physical and mental health and social well- being as well as providing critical services such as climate mitigation, endangered species habitat protection and mitigation, stormwater conveyance and moderation of flooding, air cleansing, and provision of drinking water. Community surveys indicate that Austin residents have a strong desire to conserve natural spaces for passive, unprogrammed, recreation and conservation value. Many parks vision plans specify conservation of large natural areas. Without active management, however, ecosystem health is degraded, which makes natural areas increasingly vulnerable to negative outcomes such as canopy mortality and wildfire. The 2019 City of Austin Wildfire Preparedness Audit highlighted PARD’s limited capacity to manage natural areas and recommended the creation and implementation of land management plans for high-risk areas. The purpose of this document is to guide the Austin Parks and Recreation Department in restoring and managing natural areas to mitigate risk, improve resilience, and provide ecosystem services to Austin residents in perpetuity. This guiding document will be used to inform management goals and priorities, work plans, environmental monitoring and adaptive management, implementation plans, use policies, grant applications, and departmental resource requests. Four goals guide the development of strategies to achieve the mission of mitigating risk, improving resilience, and providing ecosystems services to Austin in perpetuity: 1. Address present and emerging threats and stressors to ecosystem health that affect community-wide risk 2. Restore or mimic natural processes to support resilience and adaptive capacity 3. Restore plant community, species, and structural diversity 4. Engage the Austin community in stewardship of parkland natural areas Parkland natural areas were included in this plan based on selection criteria to identify lands most conducive to large-scale ecological restoration and management. Site-specific implementation plans will be created at the staff level in collaboration with relevant agencies and community stakeholder groups. Preliminary geospatial analyses were used to direct on-the-ground condition assessments of natural areas within the scope of the plan and process data into summary layers. A literature analysis focused on land management strategies likely to increase natural area resilience to current and future conditions and climate. Management goals and recommended management strategies are described at both system-wide and individual park scales. PROJECT CONTEXT SOCIAL EQUITY vii Historically, the City of Austin has supported policies and created structures that perpetuate racial and economic inequities. One of the effects of those policies that is still obvious today is the concentration of socially vulnerable populations east of what is now Interstate 35. Over the last several decades, research has clarified the importance of access to nature and healthy ecosystems in urban areas for human health and well-being. However, approximately 87.5% of the lands that Austin has spent public dollars to conserve lie west of Interstate 35 and approximately 98.5% of all lands that have received ecological restoration activities are located west of Interstate 35. Risk analyses in this document incorporate indicators of social vulnerability as estimates of human communities’ capacity to prepare for and recover from disaster or significant stressors such as extreme heat or wildfire or loss of access to limited public lands resulting from these stressors. CLIMATE VULNERABILITY ANALYSIS Climate vulnerability is the combination of a system’s exposure to climate change, its sensitivity to those changes, and its ability to adapt or respond. PARD lands will be exposed to increases in annual and seasonal average temperatures and are likely to experience slight decreases in annual precipitation that will fall in fewer, more intense events, as well as lower water availability and increased water stress resulting from higher temperature. Current plant community types in central Texas are projected to shift to the east and north. Climate analogues indicate that as soon as 2070, Austin’s temperature conditions will approximate those of present-day south Texas, northern Mexico, and/or suburban Phoenix, AZ. The sensitivity of PARD natural areas to these stressors will be influenced by abiotic factors such as slope, aspect, soil type, and geology and by biotic factors such as species composition, plant diversity, tree density, and canopy height and structure. Canopy mortality, intense wildfire, dramatic vegetative shifts, and novel flood dynamics are some of the likely negative outcomes of climate change in degraded systems, but these outcomes can be mitigated to a large degree by active and intentional restoration and management. Geospatial analyses mapped environmental and social vulnerability at the park scale, then merged the two to create a Park Climate Vulnerability Index. Preliminary results show a generally heterogenous pattern of vulnerability largely affected by plant community condition, soil water availability, potential for canopy fire, and social vulnerability of nearby communities. Restoration activities may be used to strategically shift the biotic factors affecting climate vulnerability and thereby reduce the risk of the negative outcomes mentioned above. LAND MANAGEMENT The following broad management goals are recommended throughout according to site conditions and conservation goals in vision plans. These goals are preliminary, to be modified as necessary as part of the adaptive management process as work plans are developed and updated and as additional data are collected. Several tracts have existing management plans. These plans were considered and incorporated, where appropriate. Of note are parklands included in the Balcones Canyonlands Preserve (BCP). These lands viii are managed under a Federal Incidental Take Permit issued by the U.S. Fish and Wildlife Service. Land management actions on BCP lands must follow Permit requirements to ensure compliance with the Balcones Canyonlands Habitat Conservation Plan (USFWS 1996) and BCP Land Management Plan. PARD will coordinate and collaborate with Austin BCP staff on management for endangered and rare species. ix Woodland Goals and Strategies x Target communitiesJuniper-oak woodland - Hardwood cover of at least 30%. Diverse species composition. Diverse age structure. Timber fuel model - open understory. Invasive species cover <25%Mixed woodlands - Diverse species composition. Diverse age structure. Timber fuel model. Invasive species cover <25%GoalsShift communities toward a Timber structure, prioritizing high crown fire risk areasReduce surface and ladder fuelsDevelop unit-level spatial heterogeneity - patch mosaic of closed canopy, low light, woodland and open woodland with diverse stand structure, age class profiles, midstory and canopy densities, and species compositionIdentify, protect, and enhance refugia - Moist, fire protected canyons, seep communities, wetlands, known populations of rare or endangered speciesSpecial emphasis on preserving healthy native trees with trunks exceeding 24" - recognized by City of Austin as heritage treesEnhance species diversity. Increase cover and diversity of fire-resistant speciesEncourage native species documented during the site assessment with moderate and low Vulnerability scores from Austin's Urban Forest and Natural Areas (Brandt et al. 2020). See Appendix 6.Introduce or encourage native species native to the Edwards Plateau or Texas Blackland Prairie with wide moisture and temperature tolerances or wide geographic ranges, with particular emphasis on heat tolerance. See Appendix 6.Manage invasive species. See Appendix 4. StrategiesMechanical thinning/fuels reductionHardwood release canopy modificationLow to moderate intensity prescribed fire and/or pile burningSpecies addition and seedling protectionGrassland Goals and Strategies Target community Grassland - Savanna or prairie structure with <30% woody cover. Diverse species composition. Goals Develop unit-level spatial heterogeneity - patch mosaic of differing prescribed fire/mowing return intervals and species composition Identify and enhance refugia - microtopography, shaded/fire protected areas Woody cover <30%. Separation of canopy between mottes. Reduction of ladder fuels particularly at boundary with woodland Increase connectivity to other grasslands within the unit Enhance species diversity. Encourage conservative grasses and forbs Encourage substantial forb cover with emphasis on wildlife value Introduce or encourage species native to the Edwards Plateau or Texas Blackland Prairie with wide moisture and temperature tolerances or wide geographic ranges, with particular emphasis on heat tolerance. See Appendix 6. Manage invasive species. See Appendix 4. Strategies Frequent prescribed fire. Varied return interval appropriate to ecosite and condition. Primarily summer and fall, occasionally winter. Range seeding in coordination with prescribed fire Mechanical thinning in coordination with prescribed fire Invasive species management xi Bottomland Goals and Strategies xii Target CommunitiesBottomland hardwood - Gallery mixed hardwood forest containing a diverse mix of obligate and facultative wetland species and high percentage of stabilizer species. Wooded riparian buffer extending approximately to the 25 year floodplain boundaryMesic grassland - Diverse grassland community with mix of obligate and facultative wetland species and high percentage of stabilizer species. Variable woody cover.GoalsSustain hydrologic processesMaintain/enhance infiltration and storage capacity in upland and bottomlandMaintain and restore floodplain connectivityMaintain and restore bottomland hardwood, grassland and wetlands. Maintain and restore vegetative cover and improve function of bottomland hardwood, grassland and wetland communitiesMaintain/enhance water qualityReduce soil erosionMaintain high vegetative cover in bottomland and riparianStructural diversityEncourage a combination of woody and herbaceous species, and diverse age classesEnhance species diversity. Increase cover and diversity of species with high stability ratingsEncourage a mixture of obligate and facultative speciesEncourage native species documented during the site assessment with moderate and low Vulnerability scores from Austin's Urban Forest and Natural Areas (Brandt et al. 2020). See Appendix 6.Introduce or encourage native species native to the Edwards Plateau or Texas Blackland Prairie with wide moisture and temperature tolerances or wide geographic ranges, with particular emphasis on heat tolerance. See Appendix 6.Identify and maintain unique habitats for refugia/ Enhance important habitats for wildlifeManage invasive species. See Appendix 4. StrategiesInvasive species managementSpecies addition and seedling protectionErosion reduction measuresMechanical thinning/fuels reductionHardwood release canopy modificationLow to moderate intensity prescribed fire and/or pile burningSpecies addition and seedling protection1 Introduction and Background 1.1 STATEMENT OF NEED Functional ecosystems are central to sustainable cities and human well-being. Natural areas provide a suite of critical ecosystem services such as climate mitigation, endangered species habitat protection and mitigation, stormwater conveyance and moderation of flooding, air cleansing, groundwater recharge, and provision of drinking water. Parklands, in particular, are vital for human health and well- being, supporting physical health, mental health, community connectedness, and social stability. Community surveys described in the Parks and Recreation Department’s (PARD) 2020-2030 Long Range Plan indicate that Austin residents have a strong desire to conserve natural spaces for passive, unprogrammed, recreation and conservation value. Many parks master plans or vision plans, which are developed with substantial community input, specify restoration or management of large natural areas. Without active management, however, the health and function of many of natural areas are degraded by such drivers as intense recreational use, loss of biodiversity, and invasive species, which makes them all the more vulnerable to climate change and wildfire. Natural areas, therefore, have the potential to, and in many cases have become, community liabilities rather than vital assets. The 2019 City of Austin Wildfire Preparedness Audit highlighted PARD’s limited capacity to manage natural areas and recommended the creation and implementation of land management plans “to reduce the threat of wildfire.” In response, PARD committed to establishing a land management program as well as draft and implement land management plans. The Austin Climate Equity Plan also recognized both the critical importance of healthy natural areas to Austin residents’ quality of life in the coming decades as well as the need to create and implement land management plans to support their healthy functioning. The purpose of this document is to guide the Austin Parks and Recreation Department in restoring and managing natural areas to mitigate risk, improve resilience, and provide ecosystem services to Austin residents in perpetuity. This guiding document will be used to inform management goals and priorities, work plans, environmental monitoring and adaptive management, implementation plans, use policies, grant applications, and departmental resource requests. 1.2 OVERVIEW OF APPROACH 1.2.1 PLAN SCOPE This Plan includes Vision and Goals, Social Equity considerations, an Ecological Framework, and a Climate Vulnerability Analysis paired with Management Recommendations intended to protect and enhance the resilience of Austin’s natural communities in light of current stressors and anticipated changes. A preliminary literature search and data collection phase identified likely climate threats which were incorporated into a desktop geospatial analysis. The desktop analysis was used to direct the on-the- ground condition assessment of parks within the scope of the plan. A second geospatial analysis was performed incorporating the results of the on-the-ground surveys. Concurrently, a literature analysis was performed focused on land management strategies likely to increase natural area resilience to the identified threats and in light of existing conditions. Results of all phases of analysis were compiled to provide a foundation for current and future land management in Austin’s parks, which are described at both the system-wide and park unit scales. 1 1.2.2 PLAN SCOPE Of the approximately 17,000 acres throughout over 300 parks managed by the City of Austin Parks and Recreation Department, 10,347.4 acres (all or pieces of 45 parks) were included in the scope of this land management plan (Figure 1-1). This acreage includes both terrestrial and aquatic areas. The scope is based on PARD’s need for a single guiding document to provide direction for achieving disparate management goals identified in numerous stand-alone plans for PARD natural areas. Site-specific implementation plans will be created at the staff level following guidance in the land management plan. PARD staff engaged in a rigorous process to select parcels for inclusion in the land management plan. The intent was to identify parkland natural areas that are most conducive to large-scale ecological restoration and management. Such large tracts are more economical to restore and manage (by taking advantage of economies of scale) and tend to provide more robust ecosystem services (primarily due to low perimeter-to-core ratios) which translate to more a favorable cost-benefit relationship with respect to expenditure of public funds and staff time. The criteria for inclusion in the scope of work: • All PARD Nature Preserves are included • All PARD lands in the Balcones Canyonlands Preserves are included • Contiguous natural areas, regardless of park type, larger than about 75-100 ac based on restoration potential and equipment access are included. For example, a 50-acre greenbelt adjacent to a 50-acre nature preserve were treated together as a single 100-acre parcel. • PARD staff consulted planning documents such as the PARD Long Range Plan as well as parks vision plans. This ensures that long-term land management planning and implementation will be consistent with the expressed needs and desires of the community and other initiatives in the Parks Department. By identifying adjacent park properties with common ecological potential and goals for park services (for example, a greenbelt adjacent to a nature preserve), staff identified large management complexes. Such management complexes enable greater ecological connectivity than individual small parcels, thus are more economical to restore and manage, have greater restoration potential, enable greater resilience, and have the potential to provide a more robust suite of ecosystem services. Natural areas that were not included in this plan are not considered to be less important for restoration or management. Rather there are other constraints such as difficulty of access and/or high restoration or management cost per acre. These tracts may be added to the land management plan at a later time once PARD has developed the capacity to address the needs of the parcels included in the current scope. These areas may also receive restoration or management conducted by volunteer groups, community organizations, or City staff using this or other plans as a guide. Examples of areas that were excluded were: • Areas that are currently developed or are planned to be developed, such as existing or future ball fields, picnic areas, mowed turf, parking lots, community gardens, or facilities, according to master/vision plans or existing conditions. Locations with high perimeter-to-core ratios (e.g. very long, narrow tracts), as these areas are difficult to access, limit the options for restoration strategies, typically require more expensive • 2 restoration strategies, typically have lower restoration potential due to high fragmentation, and thus tend to be expensive to restore and manage relative to the benefits provided. 3 Figure 1-1. PARD lands included in this land management plan. All Balcones Canyonlands Preserve (BCP) land management plans other governing documents remain in effect. 4 Table 1. PARD lands included in this land management plan. All Balcones Canyonlands Preserve (BCP) land management plans other governing documents remain in effect. 5 1.3 RELATION TO OTHER PLANS AND STUDIES Many significant plans and studies have been completed in the City of Austin relevant to land management, but most have been very high level or narrowly focused to address specific needs. Therefore, this Climate Vulnerability Analysis and Land Management Strategies plan integrates and synthesizes information collected from across the city. It provides a bridge between high-level planning and site-specific management as well as a link between city-wide fire management and natural area management considerations. Key resources that provided background for this Plan are summarized below. Several of the properties have existing land management plans. These plans were considered, and incorporated, where appropriate. Of particular note, is land managed under a Federal Incidental Take Permit, issued by U.S. Fish and Wildlife to the City of Austin and Travis County in 1996 called the Balcones Canyonlands Conservation Plan (BCCP) Permit. Land management techniques proposed on land governed by the BCCP Permit, including the Balcones Canyonlands Preserve (BCP) and BCCP cave karst fauna areas, must follow BCCP Permit regulatory requirements to ensure compliance with the BCCP Habitat Conservation Plan (USFWS 1996) and BCCP Land Management Plans. Governing documents of the BCCP Permit supersedes this plan for all affected land. References to the BCCP Permit and governing documents refer to current plans and future updates. PARD will coordinate and collaborate with BCP staff on management for endangered and rare species. 1.3.1 CLIMATE, PARK, AND SUSTAINABILITY PLANS • PARD Long-Range Plan 2020-2030, Our Parks, Our Future (City of Austin 2019): PARD's Long Range Plan is developed every 10 years and provides a blueprint to guide land acquisition, capital improvements and the development of programs and new amenities. • City of Austin Wildfire Preparedness Audit (Office of the City Auditor 2019): Part of a review of Austin’s capacity to mitigate wildfire risk, the audit report includes recommendation that PARD should ensure that land management plans are created and implemented for Parks and Recreation properties, address wildfire risk areas, and are implemented to reduce the threat of wildfire. • Austin/Travis County Community Wildfire Protection Plan (CWPP) (Bowman Consulting Group, Ltd. 2014). Austin and Travis County formed the Joint Wildfire Task Force following the 2011 Labor Day wildfires to increase the region’s wildfire preparedness and encourage development of Fire-Adapted Communities. The CWPP is the next step in developing a regional strategy to increase wildfire preparedness. The CWPP is a tool for communities and stakeholders to prepare for wildfire. The wildfire risk mitigation goals and strategies outlined in the CWPP were integrated with goals and strategies intended to enhance natural community resilience in this plan. • Austin Climate Equity Plan (City of Austin 2020): Adopted by Council in 2021, this plan sets a goal of equitably reaching net-zero, community-wide greenhouse gas emissions by 2040. Goals for natural systems include additional carbon pools, protecting 500,000 acres of farmland, reaching 50% citywide tree canopy, and neutral emissions for city-owned lands. • Urban Forest Vulnerability Assessment (Brandt et al 2020): The Austin Vulnerability Assessment is an element in the Urban Forestry Climate Change Response Framework project. It synthesizes available scientific information on both the developed and natural areas within the 6 Austin region. Chapter 5 presents an overview of management advice for nine issues: natural areas, street trees, wildlife, species diversity, municipal parks, the nursery industry, landscaping features, environmental justice, and partnerships. Tree species were also classified based on their vulnerability (in Appendix), which were incorporated into the current assessment. • Healthy Parks Plan for Travis, Bastrop, and Caldwell Counties (Austin Parks Foundation 2019): This partnership-led plan is an effort to create a shared vision for building healthier communities through parks. The plan incorporates scientific data including a heat island index, technical analysis and community engagement results to answer key questions about the people, parks and environment. • Green Infrastructure Strengths and Gaps Analysis (Siglo Group, 2021). The report includes analysis of spatial distribution of open land investments and includes evaluation of census tracts scoring lowest for health outcomes and highest for social vulnerability. Individual park vision plans including: • John Trevino Jr. Metropolitan Park o Commons Ford Ranch Metro Park o Dick Nichols District Park o o Little Walnut Creek Greenbelt o Onion Creek Metropolitan Park o Mary Moore Searight Metro Park o Roy G Guerrero Colorado River Park o Walter E. Long Metropolitan Park o Zilker Metropolitan Park 1.3.2 LAND MANAGEMENT PLANS • Balcones Canyonlands Conservation Plan (USFWS 1996): This document provides a streamlined way for landowners to comply with the Endangered Species Act, while protecting high-quality habitat in the Balcones Canyonlands Preserve. • BCCP HCP U.S. Fish and Wildlife Service (USFWS) 1996. Final environmental impact statement/habitat conservation plan for proposed issuance of a permit to all incidental take of the golden-cheeked warbler, black-capped vireo, and six karst invertebrates in Travis County, Texas. U.S. Fish and Wildlife Service, Albuquerque NM • Recommended Land Management for the Water Quality Protection Lands (Bertelsen et al 2010): Focused on source water protection, this detailed land management plan provides site- level guidance for grassland and forest management strategies. • Texas Wildfire Protection Plan (Texas A&M Forest Service 2020): Since its inception in 1915, the Texas A&M Forest Service has been tasked with the responsibility of wildfire suppression, defending both the property and lives of Texas citizens. The management perspective of the plan focuses on fire suppression and risks to property. • 2004 Memorandum of Agreement on bracted twistflower (U.S. Fish and Wildlife Service, Texas Parks and Wildlife Department, City of Austin, Travis County, Lower Colorado River Authority and the Lady Bird Johnson Wildflower Center), Note that Barton Creek supports one of the largest populations of bracted twistflowers (Streptanthus bracteatus). 7 • Land Management Plans for William H. Russell Karst Preserve Land Management Plan (COA, 2020) and Louis René Barrera Indiangrass Wildlife Sanctuary Management Plan (Siglo Group, 2020). Additionally, significant input was provided by PARD staff throughout the project to guide the process and final Plan preparation. Relevant operational plans, such as the PARD’s Integrated Pest Management Plan and the City of Austin Invasive Species Management Plan will be used as work plans are generated. 8 2 Vision and Goals 2.1 LAND ACKNOWLEDGEMENT This Land Acknowledgement was created with robust public input during the development of the Austin Climate Equity Plan (page 2, City of Austin 2020). Resources used to develop the Land Acknowledgement can be found on page 153 of that document. We wish to recognize and honor Indigenous Peoples as original stewards of the land known as Austin, Texas, and the enduring relationship that exists between Indigenous Peoples and their traditional territories. Recognizing the land is an expression of gratitude and appreciation to those whose territory we reside on and a way of honoring the Indigenous Peoples who have been living and working on the land from time immemorial. Land acknowledgments do not exist in the past tense or historical context. Colonialism is a current and ongoing process, and we need to be mindful that we are participating in it by living on colonized land. We acknowledge, with respect, that the land known as Texas is the traditional and ancestral homelands of the Tonkawa, the Apache, the Ysleta del Sur Pueblo, the Lipan Apache Tribe, the Texas Band of Yaqui Indians, the Coahuitlecan, and all other tribes not explicitly stated. Additionally, we acknowledge and pay respects to the Alabama-Coushatta Tribe of Texas, the Kickapoo Tribe of Texas, Carrizo & Comecrudo, Tigua Pueblo, Caddo, Comanche, Kiowa, Wichita, Chickasaw, Waco nations, and all the American Indian and Indigenous Peoples and communities who have been or have become a part of these lands and territories in Texas, here on Turtle Island, the ancestral name for what is now known as North America. Not all Indigenous peoples listed claim Texas as ancestral lands, as many were forcibly relocated to Texas from their ancestral homelands. It is important to understand the long history that has brought us to reside on the land and to seek to acknowledge our place within that history. The state of Texas is a product of violence carried out by colonial powers of Anglo and Mexican groups. Multiple genocides were committed on the native peoples of Central Texas as natives were hunted, detained, converted, and colonized in successive waves. Many peoples were also assimilated, including most peoples labeled Coahuiltecan and many Lipan-Apache with no treaties or recognition. Today, environmental and climate-related threats to each resident’s quality of life are inextricably linked to humanity’s long history of inequality and injustice perpetuated by legacies of colonialism and slavery, based on the exploitation of people, land, and nature. The ongoing displacement of Black, Indigenous, and communities of color on Austin’s East Side is connected to legacies of extraction of labor, theft of land, transformation of landscapes, and loss of cultures. In pursuit of resources, countries throughout the world have destroyed many ecosystems, traditional human knowledge, and interactions necessary for preventing climate change. Therefore, we need to be intentional about how we build respect for The Land and her Indigenous Peoples. The mission of the PARD Land Management Program is to restore and manage natural areas to mitigate risk, improve resilience, and provide ecosystem services to Austin residents in perpetuity. Risk refers to the potential for loss or degradation of ecosystem services, life, and property by events such as extreme 2.2 MISSION 9 heat, drought, and intense wildfire. Ecosystem services are the products and services provided to humans by natural systems. Key ecosystem services for PARD natural areas include human physical health, mental health, and social well-being as well as environmental products and services such as clean water, clean air, temperature moderation (reduced urban heat island), wildlife habitat. Resilience is the ability of a system to recover from disturbance and maintain function in the face of threats and changing conditions. Restoring resilience to Austin’s public natural areas is critical to their ability to continue serving the community throughout the 21st century as threats such as intensive use, fragmentation by development, and climate change progress. Biodiversity is a key driver of resilience, and this plan is in alignment with relevant UN Biodiversity Targets. Biodiversity is the variety of life on Earth and the natural patterns it forms. (UN Environment Program). For land managers, diversity can refer to number of species in a system (called “species richness”) and their distribution, as well as genetic variation, the number of distinct vegetative communities across a site, and/or the structural complexity (e.g., the number of vegetative layers). 10 2.3 GOALS Four goals guide the development of strategies to achieve the mission of mitigating risk, improving resilience, and providing ecosystems services to Austin in perpetuity. 1. Restore plant community, species, and structural diversity 2. Restore or mimic natural processes to support resilience and adaptive capacity 3. Address present and emerging threats and stressors to ecosystem health 4. Engage the Austin community in stewardship of parkland natural areas 11 3 Social Equity 3.1 INVESTMENT GAPS AND EQUITY MAPPING Historically, the City of Austin has supported policies and created structures that perpetuate racial and economic inequities. A detailed summary of many of these policies and their demographic and economic effects can be found beginning on page 20 of the Austin Climate Equity Plan. One of the effects of those policies that is still obvious today is the concentration of socially vulnerable populations east of what is now Interstate 35 (Figure 3-1). Social vulnerability refers to the socioeconomic and demographic factors that affect the resilience of communities, i.e. the capacity of a community to recover from highly disruptive events such as such as wildfires, floods, extreme heat events, and disease outbreaks (Flanagan et al 2011). Groups that tend to be disproportionately vulnerable include Black, Indigenous, and other people of color, low-income households, people experiencing homelessness, people with disabilities, those who speak English “less than well” such as immigrants, migrants, and refugees, and people older than 65 or younger than 17 years of age (Flanagan 2011). 3.2 NATURAL AREAS AND HUMAN HEALTH Despite dramatic technological advancement in recent decades, we as humans still, and will always, depend on healthy land and water for our most basic security and happiness. Natural areas in and around metro areas support and enhance human health and well-being in many ways that interact to reduce social vulnerability and improve resilience to disruptive events. 3.1.1 PHYSICAL HEALTH Safe outdoor spaces that encourage physical activity are correlated with better health outcomes (Mass et al 2006). City residents who live adjacent to green space tend to have lower levels of illness and disease than other people of similar income levels (Mitchell and Popham 2008) as well as higher perceptions of their own health. However, many studies indicate that there are racial, ethnic, and socio-economic disparities in access to and quality of neighborhood green space (Hondula and Barnett 2014). Those in lower socioeconomic positions are often less likely to be active than those with high socioeconomic status, in part because their neighborhood environments are often less conducive to physical activity (Chen et al 2014, Popham and Mitchell 2007). Lower income is correlated with higher risk of various conditions, including heart disease (Wolch 2014, Brunner 1997). However, income-related health inequalities are lower in populations living in areas with safe access to nature (Roe et al 2013). 3.1.2 MENTAL HEALTH Natural areas provide places and opportunities for physical activity which improves cognitive function, learning, and memory (Colcombe and Kramer 2003, van Praag et al, 1999, Pretty 2006). Outdoor activities can help alleviate symptoms of disorders such as Alzheimers, dementia, and depression (Mooney and Nicell 1992, Chalfont and Rodiek 2005, Kahn and Kellert 2002), reduce negative emotions, and increase positive emotions (Tsunetsugu et al 2013). 12 In children, nature experiences are important for encouraging imagination and creativity, improving cognitive and intellectual development, facilitating social relationships and reducing symptoms of Attention Deficit Disorder (Heerwagen and Orians 2002, Kahn and Kellert 2002, Kirby 1989). Veterans who participated in extended recreational outings in wildland settings showed increases in well-being, social functioning and life outlook (Duvall and Kaplan 2013), reduced symptoms of post-traumatic stress disorder, improvements in daily functioning (Gelkopf 2013), and improved feelings of self-control of behavior and depression (Hyer 1996). The psychological benefits of green space have been positively correlated with the diversity of its plant life (Williams and Cary 2002). People who spent time in a park with greater plant species richness scored higher on various measures of psychological well-being than subjects in less biodiverse parks (Fuller et al 2007). Time spent in nature has been shown to reduce psychological stress (Ulrich 1986, Kaplan and Kaplan 1989), particularly if initial stress levels are high (Ulrich and Addoms 1981). Walking in forests or wooded areas has been shown to produce greater physical and mental benefits than time spent in other areas (Tsunetsugu et al 2010, Morita et al 2007). Such benefits include decreased blood glucose levels in diabetics, greater immune system function (Li et al 2010), reduced levels of stress indicators (Park et al 2010). The presence of nearby natural areas may also reduce stress among those living in low-income neighborhoods (Kaplan and Kaplan 1989, Kaplan 1995). The qualities of green space are also correlated to restorative recovery (Hauru et al 2012). Parks and natural areas may contribute more positive health benefits than formalized neighborhood landscapes (Fan et al 2011). Generally, the larger the park or natural area, the greater the observed benefits (Mitchell et al 2011, Paquet et al 2013), though attention to the character and quality of the space, such as adequate sight lines to improve perceptions of safety, is important (Talbot and Kaplan 1986). 3.1.3 ECONOMICS Healthy ecosystems in and around metro areas have complex cascading economic effects throughout the community. For example, natural areas in and around cities perform a range of environmental services such as improved air and water quality, energy savings, and noise abatement. In total these services may provide billions of dollars of value each year (Nowak et al 2010). Estimated total annual removal of pollutants such as ozone, particulate matter, nitrogen dioxide, and sulfur dioxide by urban trees across 55 U.S. cities is 711,000 metric tons, representing $3.8 billion in public value (Nowak et al 2006). Urban heat island effect occurs in highly developed areas. Higher urban temperatures can raise cooling costs, discourage physical activity, and increase exposure to air pollutants such as ozone. Natural areas across a city can reduce local air temperatures by up to 9°F and dramatically reduce cooling costs (O’Rourke and Terjung 1981). Natural areas have also repeatedly been shown to improve real estate values. Homes within about one- half mile of natural areas are often valued at up to 8-20% higher than comparable properties (Crompton 2001, Hammer et al 1974, Tyrvainen and Miettinen 2000, More et al 1988). Conversely, home values have been shown to be lower near parks with a high number of users, with highly active programming 13 such as athletic events, or where the care and upkeep of developed park spaces is perceived to be poor (Luttik 2000, Schroeder 1982). 3.1.4 SAFETY People who use parks and natural areas are three times more likely to achieve recommended levels of physical activity than nonusers (Braga and Bond 2008), but perceived safety risks of these spaces can discourage outdoor activity. Although city residents value having nature nearby, they also express concern about safety based on visibility (Kaplan and Talbot 1988). In urban settings, dense understory vegetation is consistently associated with fear of crime (Fisher and Nasar 1995, Nasar et al 1993, Schroeder and Anderson 1984) because of reduced visibility (Braga and Bond 2008, Fisher and Nasar 1992). A more open understory that provides adequate lines of sight increases perceived safety in natural settings (Gobster and Wetphal 2004). This does not require a landscape devoid of understory, but rather suggests that managers should be sensitive to the relationship between vegetation density and perceived personal safety (Kuo and Sullivan 2001, Kuo et al 1998, Nasar and Jones 1997). 3.3 LAND CONSERVATION IN AUSTIN Beginning in the late 1970s and early 1980s, several initiatives related to conservation and environmental quality have been advanced in Austin. These causes have primarily centered around environmental quality and livability in residential neighborhoods, access to parks, sustaining water resources such as urban creeks, Barton Springs and the Edwards Aquifer, as well as protecting endangered species habitat and streamlining compliance with the Endangered Species Act. Land conservation has been a primary mechanism for achieving many of these goals. However, in many cities across the country, including Austin, past investments in parks and open spaces have not been equally distributed. The location of the lands that have been conserved in Austin to date is related to the geographic location of the natural resources themselves as well as to the effectiveness of various advocacy groups. This has resulted in a dramatic dissimilarity in the amount of land that has been conserved in eastern and western Travis and Hays Counties and, subsequently, in the number of Austin residents who have ready access to public natural areas. Since the early 2000s, equity mapping has become a standard approach that allows planners to acknowledge and try to correct discrepancies that arose because of structural racism, discrimination, or other factors. For example, the Community Health analysis in the Healthy Parks Plan for Travis, Bastrop, and Caldwell Counties (APF, 2019) compiles suites of indicators for both community health and socioeconomic vulnerability. Health indicators included heart disease, asthma, and obesity to identify areas with elevated need for free, nearby opportunities for physical activity. Very High priority areas tend to fall south of the Colorado River and east of Interstate 35. The socioeconomic vulnerability analysis portion of the plan included areas with low income, less than high school education, seniors and children under 5, people of color, and households without cars. East Austin had the areas with highest vulnerability, with more Very High scoring communities south of the river and near Austin-Bergstrom International Airport. The Green Infrastructure Strengths & Gaps Assessment (Siglo Group, 2021) identified stark discrepancies in the spatial distribution of open land investments. Approximately 87.5% of the lands that Austin has 14 spent public dollars to conserve lie west of Interstate 35. Few of the conserved lands east of Interstate 35 have land management plans. Further, approximately 98.5% of all lands that have received ecological restoration activities are located west of Interstate 35. The Gaps Assessment also points out that the census tracts scoring lowest for health outcomes and highest for social vulnerability had 50% more impervious surface, 50% less forest, and higher average temperatures, suggesting a role for park investments that could improve equity. The Climate Vulnerability in Austin Assessment (Bixler and Yang, 2020) also identifies the same geographies with relatively high degrees of exposure to one or multiple hazards as coupled with relatively high social vulnerability. Taken together, these data indicate a clear need for a dramatic increase in investment in natural resources and environmental quality in east Austin and eastern Travis County to reach equitable outcomes. Therefore, as part of the analysis for this land management plan, data on social vulnerability were incorporated and evaluated. Social vulnerability is incorporated into the spatial analysis for the climate vulnerability assessment described in Section 5.5. Social vulnerability refers to the capacity of communities to prepare for or recover from disruptive events such as natural or human-caused disasters or disease outbreaks. There are several robust methods for estimating social vulnerability. These include the Social Vulnerability Index developed by the U.S. Centers for Disease Control (CDC) and the Community Resilience Estimates Proportion persons 17 years or younger Proportion persons 65 years or older Proportion persons with disability 5+yrs Proportion single-parent HH with children under 18 yrs Per capita income Proportion civilian unemployed 16+yrs Proportion individuals below poverty level Proportion persons with no high school diploma 25+yrs The CDC’s Social Vulnerability Index uses 15 U.S. census tract-level variables to help local officials identify communities that may need support in preparing for hazards or recovering from disaster. Specifically, CDC uses percentile comparisons of census tracts within the state so that the rankings are comparative within a known context. These tract-level variables are: Socioeconomic Household Composition/Disability Minority status Housing type/transportation 15 Proportion HH with more people than rooms Proportion HH with no vehicle access Proportion housing 10+units Proportion mobile homes Proportion of persons who are in institutional & noninstitutional group Proportion minority Proportion persons 5+yrs who speak English "less than well" developed by the U.S. Census Bureau, and others. They are frequently used to help inform decision making, particularly with respect to public policy. All methods for estimating social vulnerability that were evaluated for use in this plan show similar results for our study area. Thus, various methods would be acceptable and valid. This plan uses the CDC Social Vulnerability Index (Figure 3-1) for ease of data access, consistency with other City plans such as the Green Infrastructure Strengths and Gaps Analysis, and recommendations from colleagues in the City of Austin Department of Public Health. 16 Figure 3-1. City of Austin’s conserved lands (Balcones Canyonlands Preserve, Water Quality Protection Lands, and parks) and 2020 Social Vulnerability Index (SVI) for Travis County. SVI data from 2020, sourced from the US Dept of Health and Human Services. 17 3.4 ENGAGEMENT AND DECISION MAKING Many of the recommendations in this document are technical in nature, but nonetheless ultimately concern human health and well-being and ecosystem services that affect local communities or user groups who, therefore, deserve a role in decision-making. Participatory decision-making and community-based planning will be incorporated to the greatest extent practicable during planning and implementation phases of restoration and land management work. Where stakeholder groups are active or can be recruited, the goal is to co-create workplans for parks or project sites and implement them in a unified fashion. This plan has benefitted from abundant stakeholder input provided during the creation of existing documents such as parks master or vision plans for properties included in this scope of work, the PARD Long Range Plan, and the Austin Climate Equity Plan. Through these robust public engagement processes, PARD has a clear understanding of Austin residents’ needs and desires with respect to healthy, thriving natural areas and the ecosystem services they provide. PARD staff also directly engaged with PARKnership groups, Adopt-A-Park organizations, conservancies, neighborhood associations, Friends groups, and other community groups relevant to each property within the scope of work. Throughout the development of this plan, approximately 50 stakeholder groups were invited to over 12 open public briefings; at least 20 community stakeholder groups and many other individuals attended. However, actively restoring and managing habitat to improve ecosystem health through treatments such as mechanical thinning, invasive species removal, and prescribed burning - as supported by scientific research and professional practice as they may be - can upset stakeholders if the treatments and goals are not well understood. Therefore, PARD is committed to engaging stakeholders at smaller, more local scales regarding habitat treatment options and planned implementation, and to manage public perception, expectation, and involvement. To accomplish these goals within local relationships, PARD has also been working to grow a community of local stakeholders at various locations through volunteer days, BioBlitzes, and educational videos detailing the ecology of various sites and their management needs. In the future, presentations within neighborhoods in the vicinity of managed natural areas are planned as connections are made and such events can be organized. Currently, there are good examples of solid partnerships between the PARD Land Management Program and local community groups involved with properties in this plan. Such parklands include Commons Ford Ranch Metropolitan Park, Blunn Creek Nature Preserve, Mayfield Nature Preserve, Red Bluff Nature Preserve, Colorado River Wildlife Sanctuary, and Stillhouse Hollow Nature Preserve. The outreach efforts of the PARD Land Management Program have been, and will continue to be, focused within communities or areas of Austin where engagement in natural resources conservation is underrepresented. In addition to growing community connection to properties in this plan, such relationships create a committed volunteer base that can be used to accomplish certain restoration treatments (i.e. harvesting seed, reseeding to increase biodiversity, manual invasive species removal, monitoring and citizen science, trail maintenance) within the scope of a broader land management program. 18 As community ties are strengthened, PARD will create the relationship infrastructure to engage local users as a parcel is considered for management activities. In-person briefings, on-site meetings or workdays, social media posts, etc., are all tools that PARD will use to engage local users regarding the needs and priorities for management treatment, the scope and timing of treatment, the post-treatment expectations, the expected long-term future conditions and benefits, and stakeholders’ active roles in the process. In time, and as relationships grow, an ambassador program could be implemented to further solidify partnerships and facilitate the land management process. As examples, the ambassador programs facilitated by both the Austin Parks Foundation and the City of Austin Office of Sustainability establish long-term and trusting relationships with community members. Compensation for service may be an important element to consider in such a program to offset the tendency of volunteer groups to be dominated by individuals who can afford to donate their time. Such a program would also need to consider the parameters of appropriate engagement and standards for volunteers as well as providing suitable tasks and training programs. Finally, such a program would need to be thoughtfully crafted and adequately funded. 19 4 Ecological Management Framework The ecological management framework is based on multiple scales including 1) ecoregional context of PARD’s lands at the system scale, 2) ecological communities (ecosites) and dynamics, and 3) unit-level conditions within the parks and complexes. Key characteristics of each of these are described here, and further details on existing conditions are in Volume 2. 4.1 REGIONAL SETTING 4.1.1 ECOREGIONS Understanding the ecoregional context of PARD’s study area is important for identifying potential ecological conditions and protection and management strategies. PARD’s parks straddle two major and distinct ecoregions: the Blackland Prairie and the Edwards Plateau (Figure 4-1). 20 Figure 4-1. Ecoregions in the study area. Source: U.S. Level III and IV Ecoregions (U.S. EPA). 21 Blackland Prairie The Blackland Prairie is a part of the Grand Prairie and runs from the San Antonio area east of what is now the Interstate 35 corridor, to the Red River (Collins et al. 1975, Diamond and Smeins 1985, 1993). It is part of the true prairie ecosystem (Weaver 1954) and as such is dominated by perennial tallgrass species, which in healthy systems is almost entirely lacking trees except along riparian corridors (Dyksterhius 1946, Weaver 1968, Riskind and Collins 1975). Tallgrass prairies once dominated the region, shaped by frequent fire and periodic bison grazing. Natural fires and infrequent, but intense, grazing and animal impact suppressed woody species growth and encouraged the dominance of grasses and forbs (broadleaf herbaceous plants). An intact remnant of the Blackland prairie in Round Rock has been documented as having over 200 species (Gee and Campbell 1990). Researchers have documented at least six distinct vegetative communities making up the Blackland prairie (Collins et al. 1975), but the native tallgrass prairies of the Blackland Prairie ecoregion are now extremely rare. The dark soils are primarily Vertisols, with high clay content that shrinks when dry and swells when wet, causing significant soil movement. This shrink/swell pattern contributes to the development of microtopography. Alfisols are present as well and support a different community due to their sandy loam texture. Small depressions and rises called Gilgai and mima mounds form and provide habitat diversity on a microscale, allowing different plant communities to develop within 22 feet of each other. Gilgai are shallow depressions and Mima mounds are small circular hills. These microtopographical features increase water capture significantly (Griffin et al 2007). Sometimes prairies were impassable after rain because thousands of gilgai held pools of standing water (Hayward and Yelderman 1991). The immense infiltration capacity allowed the prairies to capture and clean the water from all but the most intense storms, and streams and runoff were clear. Common prairie grasses include little bluestem (Schizachyrium scoparium), big bluestem (Andropogon gerardii), yellow Indiangrass (Sorghastrum nutans) and switchgrass (Panicum virgatum). Lowlands contain eastern gamagrass (Tripsacum dactyloides) and switchgrass. Common forbs include asters (Aster spp.), prairie bluet (Hedyotis nigricans), prairie clovers (Dalea spp.), and coneflowers (Rudbeckia spp.) Forested areas were historically concentrated areas along streams and within scattered clusters of upland trees. Common bottomland species include bur oak (Quercus macrocarpa), Shumard oak (Quercus shumardii), sugar hackberry (Celtis laevigata), elm (Ulmus spp.), ash (Fraxinus spp.) eastern cottonwood (Populus deltoides) and pecan (Carya illinoinensis). Following settlement by Euro-Americans in the late 1800s, the historic prairies were rapidly lost. The region’s soils made it popular for farming in the late 1800’s and early 1900’s and most of the prairies were plowed for agriculture. Most were converted to cropland or non-native pasture of introduced grasses such as Johnsongrass (Sorghum halepense) bermudagrass (Cynodon dactylon) or King Ranch bluestem (Bothrichloa ischaemum). This change increased soil loss through erosion dramatically. It also changed the health of the soil. A grassland’s biomass is largely underground, in a complex community that builds soil, cycles nutrients, and supports infiltration capacity. The new croplands did not do this, and the prairie soils degraded as result. More recently, former prairies are being put to urban uses and most of the prairies have been converted to agricultural or urban uses. Today, essentially all gilgai and mima mounds in central Texas have been destroyed by tillage or earth- moving, although they may naturally reform over long time periods. Hydrologically, losing the dense tallgrass community and the water-trapping gilgai reduces water infiltration, increases runoff and soil loss, and further degrades wildlife habitat that has been altered by the change in plant communities (Griffith et al. 2007). The Blackland Prairie’s open, flat, nature also allowed clearing and agriculture all the way up to the waterways. The forested communities that once existed along waterways stabilized the bank and their loss has contributed to stream incision and instability as well as loss of water quality within the region (Olinde et al 2021). The Texas Blackland Prairie ecoregion is one of the most critically threatened ecoregions in the state, with less than 1% remaining in good condition (TPWD 2012). Within the Blackland Prairie, active restoration will be needed for upland, bottomland, and riparian communities in the project area to address significant challenges such as loss of species diversity and native seed banks (Olinde et al. 2021), vegetation type conversion, shrub encroachment, interruption of natural disturbance process, and dominance of invasive species. 23 Edwards Plateau The Edwards Plateau is a dissected limestone plateau crossed by a sparse network of perennial streams. Plant communities are distributed along moisture and topographic gradients. The Balcones Canyonlands forms the southern and eastern border of the Edwards Plateau. Mesic riparian woodlands are found along waterways. Juniper-oak woodlands dominate exposed slopes. Loss, natural absence, or reduction in frequency or intensity of the processes that maintain open grassland result in a more closed canopy. The region’s predominantly Mollisol soils also vary along a topographic gradient (Figure 4-5). Soils tend to be shallower on hillslopes and deeper in the valleys and on flat ground. Ashe juniper (Juniperus ashei) and mesic species such as escarpment black cherry (Prunus serotina spp. exima), little walnut (Juglans microcarpa), rusty blackhaw viburnum (Viburnum rufidulum) are dominant in areas with naturally shallow, rocky soils, in moist areas such as sheltered canyons, or in areas protected from natural fire such as narrow mesas bordered by canyons. Riparian areas include bald cypress (Taxodium distichum), American sycamore (Platanus occidentalis), black willow (Salix nigra), red buckeye (Aesculus pavia), boxelder (Acer negundo), and buttonbush (Cephalanthus occidentalis). The prevalence of Ashe juniper (Juniperus ashei) has likely increased in many areas since the late 19th century, discussed further below. It can be difficult to distinguish juniper-invaded habitats from areas where juniper was a naturally dominant component. Additional common tree species in the Austin area include Texas oak (Quercus buckleyi), plateau live oak (Quercus fusiformis), Texas persimmon (Diospyros texana), and cedar elm (Ulmus crassifolia). Threats to these communities include fire suppression, wildfire, livestock grazing, invasive species, damage by feral hogs and vehicles and timber clearing. 24 Grasslands include little bluestem, yellow Indiangrass and sideoats grama (Bouteloua curtipendula). In heavily-grazed areas, Texas wintergrass (Nassella leucotricha), and threeawns (Aristida sp.) are more common. This is a karstic landscape with surface water and groundwater closely connected as rain and streamflow infiltrates sinkholes, fissures, and caverns of the limestone substate recharging the Edwards Aquifer and supplying springs. Partly because of this complex geology and rough terrain, the region supports a diversity of communities and species and is considered a biodiversity “hot spot”. Species endemic to the region include the widemouth blindcat (Satan eurystomus), Comal blind salamander (Eurycea tridentifera), Jollyville Plateau salamander (Eurycea tonkawae), Barton Springs blind salamander (Eurycea sosorum), Austin blind salamander (Eurycea waterlooensis), and Blanco blind salamander (Eurycea robusta). Mexican free-tailed bats (Tadarida brasiliensis) use the caves as maternity roosts. The endangered golden-cheeked warbler (Setophaga chrysoparia) nests exclusively in mature oak-juniper woodlands in central Texas while the recently de-listed black-capped vireo (Vireo atricapilla) nests in dense, mixed shrublands across central and southwest Texas. (Griffith et al. 2007) Historic disturbance and land cover patterns Fires, intermittent grazing, the region's varied topography and soils, and drought-prone, subhumid tropical climate created a mosaic of woodlands, forests, and grasslands. The historic fire regime and regional woodland cover is debated, but woodlands likely covered approximately half of the total land area and most of the slopes (Diamond and True 2008, Bray 1904, Reemts and Hansen 2013). Juniper-oak woodlands were the most common type of woodland in the uplands with deciduous hardwoods becoming more common in bottomlands and along drainages. The two dominant species, Ashe juniper and Plateau live oak (Quercus fusiformis), have different responses to fire. Plateau live oak resprouts vigorously following fire. Conversely, Ashe juniper does not have the ability to resprout following top- kill, thus small statured juniper trees are frequently killed by low-intensity fire, but even very large juniper trees can be killed by more intense fire. Seedlings and saplings of both species are sensitive to fire. Fire tends to burn poorly, if at all, in areas of thin soils that support weak grass growth as well as in sheltered canyons and drainages. Thus, woodlands of fire-sensitive species tend to develop in these locations. Areas of generally flatter topography and deeper soils that support continuous grass growth tend to facilitate frequent fires and promote savanna (Bray 1904, Diamond et al. 1995). Bray (1904) noted that some of the canyons had very old trees, indicating how long forest development had been occurring. Bray noted that until near the time of his account, timber in the canyons and hills gave way to treeless grassland on the level plateaus. Several types of woody communities were supported, influenced by moisture availability (Table 2). Canyons, with high water availability supported taller trees and well-developed forest. Trees gradually thinned and grew smaller through the wooded communities of the hills and bluffs, finally giving way to grassland on the flats. At the time, the vegetation of the region was undergoing change. Woody species were expanding into former grassland while old-growth forests were logged for posts and lumber. Woodlands dominated by fire-tolerant species such as live oak occasionally may have established in the flatter plains and mesas where the stochasticity (i.e., random patterns) of weather and fire events resulted in fire return intervals long enough for establishment. Live oak mottes within a grassland matrix likely persisted for hundreds of years (Jessup et al. 2003) as the interiors effectively exclude 25 intense fire, live oaks are resistant to low intensity fire, and resprout vigorously after intense fire. Juniper breaks also effectively exclude fire under most conditions, however, fires were noted to be frequent in dry Ashe juniper stands on the uplands (Bray 1904). It was noted that after a cedar brake burned, it grew back as “a different kind of brush” described as oak shinneries (dense shrublands) (Diamond et al. 1995). Much of the recent debate surrounding juniper woodlands in the Edwards Plateau extends from this dichotomy – juniper woodland simultaneously contracted (via clearing) and expanded (via fire suppression) in different areas. Old growth juniper woodlands were largely harvested and few remain. Historically, juniper can and did establish in open savanna but was periodically reduced again by fire. Land use patterns changed with Euro–American settlement. Overgrazing and fire suppression in the grasslands encouraged woody species expansion into these areas. Drought has been, and continues to be, an important influence in the region. Woodland composition can change dramatically following extended drought. The drought of the 1950s took a heavy toll on woody plants in the Edwards Plateau with large Ashe junipers suffering 90% mortality, but junipers <2m tall experiencing little mortality (Diamond et al. 1995). Little leaf shin oak mottes (likely Quercus vaseyana) were also reported to have died during the drought. A more recent study documented a 22% decrease in post oak (Quercus stellata) following the 2010-2011 drought (Bush and Van Auken 2018). 26 Table 2 Major pre-European savanna and woody forest community types with Ashe juniper as an important component in the Central Texas Hill Country (Excerpted from Diamond 1995). Community Type Plateau Live Oak-Ashe Juniper Ecological Site Type Community Characteristics flat or rolling uplands; fairly deep, continuous soil savanna or woodland with individuals or mottes of oak; Ashe juniper and other tree species in mottes Post Oak/Blackjack Oak-Ashe Juniper flat or rolling uplands; reddish, relatively deep soils over chert-rich limestone savanna to well-developed woodland; Ashe juniper and other tree species in dense mottes or better developed woodlands Scalybark Oak/Vasey Oak (west)-Ashe Juniper Ashe Juniper- Texas Oak/Deciduous Tree Species flat or rolling uplands, low upland scarps, or canyon rims, soils shallow or discontinuous over massive fractured limestone slopes, canyons, escarpments, and creek sides shrubland or woodland depending on fire frequency or recovery time post crown fire; Ashe juniper increasing with time since previous burn evergreen woodland (dry exposures/dry slopes) to mainly deciduous forest (wet exposures, seeps, creek-sides); vegetation forming horizontal bands on slopes Fire Frequency/ Type Woody Species Diversity high; surface fires with few crown fires high to medium; few crown fires medium to low; crown fire as at decades- long intervals low; fire absent or crown fires after drought or at long intervals low medium medium to high high 27 Historic community descriptions (based on Bray 1904) • Bottomland/riparian forest. Along rivers, gallery forest often developed with three of four strata, dense canopy and shade adapted species underneath. Species include Bald cypress (Taxodium distichum), American elm (Ulmus americana), sycamore (Platanus occidentalis), pecan (Carya illinoinensis), overcup oak (Quercus lyrate), basket oak (Quercus michauxii), cottonwood (Populus deltoides), Texas red oak (Quercus buckleyi), and hackberry (Celtis occidentalis). Most of the trees here become tall. Midstory species include black cherry, box elder, walnut, and soapberry. • Hill and bluff timber. This is the dominant woodland type in the region. Density varies with local conditions. Lower flats with darker soil support heavy mixed woodland of cedar, live oak, cedar elm, hackberry, shin oak, and what Bray called “mountain oak,” a term for several species of low-growing oak. • Shinneries. Found on areas with very thin soils above limestone. Mixed species, but primarily dense thickets of short-statured oaks such as Shinnery Oak (Quercus havardii). • Cedar brakes. Ashe juniper dominated, very dense thickets found on xeric limestone slopes. Other species grow within the juniper. After juniper is eliminated for a time following crown fire, the cedar brakes often recover to shinneries as the species previously comingled with, but suppressed by, the juniper resprout in the absence of competition. • Post oak timber. Primarily found in sandy or gravelly soils. Post oak dominate but blackjack oak are common in an open, park-like growth. On rich and moister sandy soils, post oak could reach large size with a dense undergrowth. Edwards Plateau Community Distribution Today The distribution of woodlands and grasslands across the Edwards Plateau is summarized in Table 2. Currently, woodland slopes are twice as common as grassland slopes. High flats are more evenly divided between woodland and grassland, and low flats and floodplains support more woodland (Diamond and True 2008). Table 3. Abiotic site types were summarized based on analysis of 30-m resolution digital elevation models and then overlaid the 30-m resolution satellite-derived land cover such as the national land cover database. Adapted from Diamond and True 2008. Abiotic site type Woodland (%) Grassland (%) Urban (%) High Flats 50.1 45 Slopes 68 Low flats and floodplains 47.7 29.8 36.1 4.8 2.1 16.2 28 4.1.2 GEOLOGY The project area is broadly divided between sedimentary, carbonate formations to the west and sedimentary, clastic and undifferentiated formations to the east (Figure 4-2). Surficial geology on the east side of the project, underlaying the Blackland Prairie, is Holocene and Pleistocene sand, silt and clay, while bedrock geology is Miocene, Oligocene, and Eocene sandstone and claystone (Griffith 2007). The Upper Cretaceous marine chalks, marls, limestones and shales give rise to the characteristic black, calcareous, alkaline and heavy soils (primarily Vertisols) (Griffith 2007). Figure 4-2. Geology of the study area. Source: State Geologic Map Compilation (SGMC) geodatabase of the conterminous United States (2017) Geology on the western side of the project, underlaying Edwards Plateau is more complex. Information presented here, unless otherwise noted, comes from the Barton Springs/Edwards Aquifer Conservation District’s description of the Recharge Zone (refer to Figure 4-4) or the Texas Cave Management Association. The geologic units that comprise the Recharge Zone are the seven members of the Edwards Limestone and the Georgetown Formation. The Marine member is present only in the southern portion of the Recharge Zone. The Leached/Collapsed member outcrops over large areas along the eastern edge 29 of the Recharge Zone and contains many caves. The Regional Dense member acts as a confining unit and concentrates water flow, which promotes cavern development. The Grainstone member is a relatively massive unit that protects the numerous caves that occur in the underlying Kirschberg member. The Dolomitic member is not as prolific a cave-forming member but contains larger sized passages due to the nature of the bedrock. The Walnut member is a very marly limestone, i.e. limestone with a large clay content. This member is not a good cave-forming layer. Beneath the Edwards is the Glen Rose limestone, a relatively impervious member that is hydrologically connected to the Edwards Aquifer. The fracturing along and adjacent to the numerous small faults along the eastern edge of the Recharge Zone tend to increase the amount of recharge and thus the sensitivity of this area (Russell and Jenkins 2001). Cave and karst systems are important for two major reasons. First, the overwhelming majority of the nation's freshwater comes from groundwater. About 25% of the groundwater is located in cave and karst regions. The protection and management of these vital water resources are critical to public health and to sustainable economic development. As identified by the National Geographic Society, water resources are a critical concern as our society enters the twenty-first century (Kerbo 1998). Note, that assessing the vulnerability of the caves and related resources in Austin was outside the scope of the current project, but it is a topic that merits further study in the future. 4.1.3 HYDROLOGY Most of the study area is within the major Austin-Travis Lakes watershed, with the exception of the Decker-Barrera-Long park complex located in the more easterly Lower Colorado-Cummins watershed (Figure 4-3). Several of the significant waterways traversing the parcels in this plan include Barton, Onion, Slaughter, Walnut, Marble, and Decker Creeks. 30 Figure 4-3. Watersheds of the study area. Watershed boundaries delineate subbasins that drain to a portion of a stream network. Hydrologic unit codes (HUCs) designations 8 and 12 refer to regional and local scales, respectively. Source: USGS National Hydrography Dataset (NHD). 31 Figure 4-4. Major aquifers in the study area. The Edwards and Trinity Aquifers -- two of Texas’ nine major aquifers - are found in the study area. Aquifer outcrops, or recharge zones, are present in the west where the formations are exposed at the surface, whereas the aquifers are buried in the subcrop areas in the east. Source: TWDB, December 2006. Approximately 25% of the parks overlie outcrops of the Edwards or Trinity Aquifers on the west side of the study area. (Figure 4-4) Surface water and ground water connections exist across the study area and vary by rock type, position in the watershed, and ecological sites. The Edwards Plateau region has karst topography with fractured bedrock and a sandy substrate, which allows surface flow to rapidly become stored groundwater (Wilcox 2010). A recent creek flow loss water balance (Hauwert 2016) utilized groundwater tracing results to directly delineate water sources to Barton Springs, added flow stations tied to the mapped groundwater basins. This water balance measured major stream channel recharge contribution of 56 to 67% of total groundwater discharge (including well discharge) in groundwater basins feeding Barton Springs. An estimated 17% of creek channel recharge originated from upland recharge zone areas between the major creeks. Upland Edwards Aquifer Recharge and smaller sources such as inter-aquifer flow contributed 33% to 44% of total groundwater discharge. As a percent of rainfall, an average 22% to 28% 32 of rainfall originating from the Edwards Aquifer recharge zone recharged the aquifer in comparison with climate tower average measurements of 28% of rainfall (Hauwert, 2014). Rainfall plays a significant role in stream hydrology, with flow depth across the area changing relatively quickly in response to large, rare rain events such as the Halloween Flood of 2015. Larger events shape the larger scale valleys and terraces. Smaller, frequent, 2-year rain events shape the primary flowpath we see during bankfull flow. These events are ecologically and geomorphically important. Vegetation and land cover throughout a watershed has a significant effect on surface flow, river baseflow, bank stability and as water quality because they determine infiltration, runoff and soil erosion ratesThe Blackland Prairie has experienced significant loss of riparian and bottomland vegetation as well near complete replacement of upland prairies. The loss of prairie systems capable of capturing and cleaning stormwater had increased the amount of runoff reaching waterways and increased its sediment load, while the loss of riparian and bottomland woodland has reduced stream stability. The stability of Blackland Prairie streams is further compromised by the lack of hard points such as bever dams, grade control via debris dams or roots that existed historically in the region. The absence of these features, combined with the fine soil of the region contributes to unstable waterways (Olinde et al. 2021). The Edwards Plateau has retained more of the natural bank and upland vegetation but has also experienced losses to overgrazing and urbanization. The interrelationships of hydrology and vegetation are discussed further in Section 6. 4.1.4 SOILS Soil type is characterized based on the general slope of the area, composition of the parent material, and subsequent percentages of clay, silt, sand and rock in the soil. Five dominant soil orders are found in the study area (Figure 4-5). As previously noted in the ecoregional descriptions, soils underlying the Blackland Prairie in the east are primarily Vertisols with high clay content, with occasional Alfisols. Soils underlying the Edwards Plateau in the west are primarily Mollisols and Inceptisos. Soil types that have historically supported similar vegetation communities are grouped into ecological sites. Each ecological site description (see also Section 4.2 and Appendix 7) includes the average annual biomass production, an assessment of its suitability for grazing, the historical climax plant community, the plant species that appear and disappear under heavy grazing and the huntable wildlife native to the area. A useful analysis of the current condition of a given piece of land can be obtained by comparing the current assemblage and biomass of species to the historical climax system described in the ecological site description. The Natural Resources Conservation Service (NRCS) has mapped 85 soil units (at a finer scale than the soil orders noted above) which are grouped by series within the study area. Of these, Speck, Eckrant, Brackett, and Houston Black soil series make up 47% of the total area. Most of the soils on the Edwards Plateau consist of Speck clay loam (1 to 5 percent slopes). On the Blackland Prairie, the soil mostly consists of Ferris-Heiden complex (8 to 20 percent slopes). 33 Figure 4-5. Soils of PARD lands. Natural Resource Conservation Service (NRCS) identifies five dominant soil orders in the park lands -- mollisols, vertisols, inceptisols, entisols and alfisols in order of abundance -- which refer to their different formations and characteristics. 34 4.1.5 PLANT COMMUNITY TYPES There are five general land cover types included in the PARD study area -- juniper woodland, mixed woodlands, grasslands, bottomland, and developed lands (Figure 4-6). For this Plan, these cover classes are further subdivided into more specific plant community types as described in detail in Section 4.2 Ecological Site Descriptions and Section 6. Figure 4-6. Dominant plant community types in PARD lands. Community types derived from site assessment performed as part of this project. 35 4.1.6 HABITAT General habitat areas for multi-species have been mapped at regional scales across Travis County as shown on Figure 4-7. Areas with “Recognized biodiversity value” relative to national landscapes are identified by The Nature Conservancy (Anderson et al., 2018). ESRI has also developed high-level national data as part of their Green Infrastructure Initiative to assist planners with preliminary connectivity considerations at multiple scales. Additional discussion of habitat characteristics in the study area are provided in Sections 4.2 and 6. Figure 4-7. Habitat areas in the study area. National datasets identify areas of recognized biodiversity, general habitat cores (ie not species specific), and connectivity importance for conservation planning. 36 4.1.7 LAND USE Recent trends in land use in Travis County and Austin have been dominated by the rapid and expansive growth of the region for new development. That said, the two large ecoregions of Austin face slightly different conditions. Most broadscale challenges affect both, but it is worth noting that the threats to PARD natural areas include localized challenges to sensitive habitats, such as vernal pools, forested wetlands, cliff face/seep communities, older growth forest remnants, or sites with threatened and endangered species which cannot be generalized and are discussed further in Section 6. 4.2 ECOLOGICAL SITE DESCRIPTIONS The NRCS classifies all lands in the U.S. into distinctive “ecological sites” based on common soil, historic vegetation, and ecological drivers (USDA 1997). The ecological site classification system provides a consistent framework for classifying and understanding forest and rangeland soils and vegetation, thereby delineating land units that share similar capabilities to respond to management activities or disturbance. Ecological sites are commonly utilized as the basic subdivision for inventory and analysis of natural areas. For each ecological site, the NRCS descriptions identify possible states according to current and historical properties of soil, hydrology, and landscape position. Inventory and analysis efforts use knowledge of these individual ecological sites and their interrelationships to one another on the landscape to develop management decisions. The project area contains 19 ecological sites, though only 16 cover more than 20 acres. For the purposes of informing land management decisions, conceptually many of these ecological sites follow similar transitional pathways between woodland, grassland, and savanna states, as will be described here. Woodland descriptions within the ecological site descriptions for the City of Austin lands are limited, so we have drawn on NatureServe Alliance descriptions to supplement our understanding of this community type. Attributes of each site that are useful to informing management decisions are called out in Section 4.2.2, and the complete Ecological Site Descriptions can be found in Appendix 7. 4.2.1 STATE AND TRANSITION PATHWAYS Community dynamics are driven by soil, moisture, topography, and disturbance regime. State and transition models identify the most common potential ecological states that are possible on each ecological site as well as the land use drivers that cause transitions between states. State and transition models are used by land managers to understand strategies and actions that may help achieve management goals. Communities within the project area have the potential to shift between grassland (prairie and savanna), shrubland, woodland and converted land states. Converted land has been used for cropland or some other use that has eliminated the native community and damaged or significantly altered soil structure. Grassland states are maintained by repeated fire that keeps coverage of woody canopy low. Periodic, intense grazing followed by long rest periods help maintain species and structural diversity and reduce the accumulation of heavy thatch. As fire frequency declines, woody cover increases. Prairies are considered to have crossed into savanna states when woody cover lies between 5 and 20% cover. Prairie and savanna states cross a threshold 37 into a shrubland or woodland state once woody cover exceeds about 30%. Depending on soil type, common encroaching woody species include juniper (Juniperus sp.), live oak (Quercus virginiana), cedar elm (Ulmus crassifolia), mesquite (Prosopis glandulosa), and hackberry (Celtis spp.), and an assemblage of shrubs. If this shift is accompanied by continuous, rather than intermittent grazing, changes in the herbaceous community will occur. Under continuous heavy grazing, conservative tallgrass species are typically lost first. These species are referred to as “decreasers” because they decrease in abundance with grazing intensity. This includes many species that historically dominated tallgrass communities such as big bluestem (Andropogon gerardii), Indiangrass (Sorghastrum nutans), switchgrass (Panicum virgatum), eastern gamagrass (Tripsacum dactyloides), and ultimately little bluestem (Schizachyrium scoparium). The community then becomes dominated by often unpalatable mid-grass species referred to as “increasers.” Common increaser species include Texas wintergrass (Nassella leucotricha), Texas grama (Bouteloua texana), three-awn (Aristida sp.), and dropseeds (Sporobolus spp.). Under further continuous, heavy grazing, grasses may cease to dominate the herbaceous community and may be replaced by grazing-resistant and disturbance-tolerant forbs, or in extreme cases bare ground. Palatable species will be replaced by unpalatable species such as Texas wintergrass, nightshade (Solanum sp.), broomweed (Amphiachyris dracunculoides), and prickly pear cactus (Opuntia sp). Perennial species begin to give way to annuals as well and the wide variety of forbs that characterized the reference community is generally reduced. A substantial portion of the project area is in the woodland state, which is poorly described in the ecological site descriptions. The Ashe’s Juniper (Juniperus ashei) Woodland Alliance and The Texas Live Oak - Ashe’s Juniper (Quercus fusiformis - Juniperus ashei) Woodland Alliance (NatureServe 2023) describes a significant proportion of the upland woodlands that currently exist within the project area within the Edwards Plateau. Within the Blackland Prairie, upland woodlands are often dominated by mesquite (Prosopis glandulosa) encroaching grasslands and can be described by the Honey Mesquite Scrub Woodland Alliance. Many of the project’s woodlands within the Blackland Prairie are secondary successional old fields described by the, the Sugarberry – Elm (Celtis laevigata – Ulmus) Forest Alliance and the Ashe Juniper Woodland Alliance. The Plateau Live oak – Ashe Juniper (Quercus fusiformis – Juniperus ashei) Woodland alliance is also common. Many Blackland Prairie woodlands within the project are found in drainages and can be described by Pecan-Cedar Elm – Sugerberry (Carya illinoinensis – Ulmus crassifolia – Celtis laevigata) Flooplain Forest Alliance. Some Bald Cypress – Sycamore (Taxodium distichum – Platanus occidentalis) type Floodplain Forest Alliances exist in both ecoregions. Hydrology and State Transitions Vegetative cover, composition, and condition all influence how water moves through the landscape and how effectively water infiltrates into the soil profile. Under native grassland, for example, infiltration is rapid due to high soil organic matter, good soil structure, and high porosity. Generally, neither rills nor gullies have formed within the community. Infiltration under woodlands is good, improving as woodlands mature and add more organic matter to soil. Woodlands also intercept a portion of annual rainfall in their canopies, a significant portion of which is then lost to evaporation (Thurow et al. 1987, Thurow and Hester 1997, Hicks and Dugas 1998, Schuster 2001, Owens and Lyons 2002, Owens et al. 2006). 38 State transitions also have important implications for hydrology. Improper grazing can reduce ground cover, resulting in a transition to a degraded community. This negatively impacts the natural hydrology as infiltration declines and runoff increases due to poor ground cover, low organic matter, erosion and poor structure. Combining sparse ground cover with intensive rainfall also creates conditions that increase the frequency and severity of flooding. In extreme events, streambank stability will decline and erosion of waterways will increase. Trees and shrubs increase the infiltration capacity of the soil beneath them. Tree litter and windblown sediments accumulate beneath woody species, increasing soil organic matter, microbial activity, and porosity (Dunkerley 2000), and surface runoff under healthy woodlands is low (Hester et al. 1997). Increased infiltration can lead to enhanced baseflow and water yield (Jones et al. 2022). Wilcox and Haung (2010) demonstrated that stream baseflow in the Edwards Plateau has been increasing since the 1940s along with woody community cover, arguing that improved community condition in woodlands and grassland following reduction of overgrazing in the 1960s accounts for the change. 4.2.2 ECOLOGICAL SITE SUMMARIES The following characteristics help distinguish common ecological sites when planning for target communities and desired outcomes of land management. ● Historic climax community: Tallgrass prairie ● Deep soils, predominantly Vertisols, often poorly drained ● Primary invasive species: Johnsongrass, Bermudagrass, giant cane, King Ranch bluestem ● Primary encroaching woody species: taproot species such as honey mesquite and hackberry, eastern red cedar Ideal prescribed fire return intervals are short (2-4 years) due to rapid woody encroachment ● ● Historic climax community: Tallgrass prairie ● Similar to Blackland, but soils tend to be more well drained ● Located primarily in alluvial plains, such as near the Colorado ● Historic climax community: Mixed-grass prairie, live oak savanna ● Located predominately on Edwards limestone geology within the Barton Springs segment of the Edwards Aquifer recharge zone. ● Karst formation. ● Very well drained with high water yield (runoff + recharge). Significant for aquifer recharge and water supply. ● Primary invasive species: King Ranch bluestem, Malta star thistle, bastard cabbage ● Primary encroaching woody species: Ashe juniper, live oak, cedar elm ● Ideal prescribed fire return intervals are moderate (3-7 years) Blackland Clay Loam Redland 39 Low Stony Hill ● Historic climax community: Mixed-grass prairie, live oak savanna ● Similar to Redland but soils are shallower and more variable ● Primary encroaching woody species: Ashe juniper, live oak, cedar elm, dense shrubs such as ● yaupon and mountain laurel. Ideal prescribed fire return interval is short (2-4 years) to moderate (3-7 years) depending on density of resprouting shrub cover Adobe sheltered sites ● Historic climax community: Mixed-grass prairie, live oak savanna, oak-juniper woodlands on ● Vegetation is unique and variable. Potential for mixed grass oak savanna to dense shrubland to old growth juniper/oak woodland depending on exposure to fire, topography, and moisture regime. ● Historic vegetation in the canyons or in other fire-sheltered areas was likely old-growth oak- juniper timber. ● Low soil organic matter and xeric conditions lead to low invasive species cover in many areas, with a high proportion of herbaceous species likely adapted to expected climate change conditions. King Ranch bluestem may invade in low areas and along stream channels where higher organic matter soil accumulates. ● Herbaceous vegetation community is unique in the Austin area dominated by little bluestem and mid-grasses such as three awn and many other species not commonly found elsewhere in the area. Grasses include: tall gramma (Bouteloua hirsuta), slim tridens (Tridens muticus), hairy tridens (Erioneuron pilosum), seep muhly (Muhlenbergia reverchonii) on the slopes, big muhly (Muhlenbergia lindheimeri) in the bottoms. Common forbs include: blackfoot daisy (Melampodium leucanthum) and several others. ● Topography is the steepest in the area with stair-stepped hillsides, flat plateaus, and steep canyons typical of the Glen Rose formation. Historic vegetation was likely a function of topography. Canyons had higher moisture availability and were sheltered from fire allowing complex, old growth, woodlands to develop. Plateaus had more fire exposure. ● Glen Rose geology has slower groundwater recharge than Edwards geology. The Glen Rose outcrop recharges the Trinity Aquifer. ● Low soil organic matter and xeric conditions lead to slower successional rates which allow for longer fire return intervals (4-10 years) to maintain grassland. Vegetation and soils are more sensitive to compaction and grazing than other site descriptions. ● Historic climax community: Tallgrass savanna or gallery forest ● Occurs primarily within the Blackland Prairie ecoregion ● Current communities are almost all woodland, though wide floodplains were likely a mix of open woodland and tallgrass communities historically. ● Primary canopy species are taproot species suited to deep soils and short-term flooding. Common species include pecan, cottonwood, American elm, hackberry, and box elder. Pecan woodlands likely had an open understory with continuous grass-dominated herbaceous layer. Bottomland 40 Gravelly ● Historic climax community: Tallgrass/Oak savanna ● Minor portion of project area, but it is associated with post oak savanna. Post oaks occur predominately in sandy or gravelly soils such as alfisols, thought they may occur on well-drained molliols as well. 41 5 Climate Vulnerability Analysis Climate vulnerability is the combination of a system’s exposure to climate change, its sensitivity to those changes, and its ability to adapt or respond. This chapter begins with 5.1 Climate Change Summary to provide a brief characterization of exposure in terms of expected future climate conditions based on accepted City of Austin planning documents. Sensitivity is described in 5.2 Ecosystem Components, with a focus on the abiotic and biotic factors that make areas more sensitive or conversely more able to adapt. The future of Austin’s ecological systems relative to other geographies is discussed in Section 5.3 Climate Analogues. Section 5.4 Climate-driven Natural Area Outcomes describes the most concerning consequences that natural areas will experience based on the expected future conditions, considering relevant effects of exposure to biological systems. Finally, Section 5.5 Geospatial Analysis pulls together exposure to major climate drivers and ecosystem sensitivities to synthesize and map Austin parks’ vulnerabilities. Taken together, these changing dynamics represent an interruption of natural processes that interacts with human uses, particularly in intensive visitor use areas, to reduce an ecosystem’s structural and species diversity. This loss of complexity impairs ecosystem functions and increases the risk of significant negative events such as widespread canopy mortality and wildfire. 5.1 CLIMATE CHANGE SUMMARY 5.1.1 CO2 CONCENTRATIONS AND EMISSIONS SCENARIOS The pre-industrial atmospheric CO2 concentration was approximately 280 parts per million (ppm) (IPCC). In 2010, it reached 400 ppm – a 43% increase. Most predictive climate models use estimates of future greenhouse gas (GHG) emissions based on various mitigation scenarios. These models calculate the effect of GHG concentration in the atmosphere on the balance of heat entering and leaving Earth’s atmosphere. The change in this balance of heat is called radiative forcing. The various patterns of change in GHG concentration over time as a result of various emissions scenarios are called Representative Concentration Pathways (RPCs) and are labeled according to the amount of radiative forcing they are estimated to cause by the year 2100. Common RPCs, with the unit watts per square meter (W/m2), used in climate modelling are RPC 2.6 for a low emissions scenario, RPC 4.5 for moderate emissions, and RPC 8.5 for high emissions. These RPCs would lead to 450 ppm, 550 ppm, and 936 ppm CO2, respectively. 5.1.2 TEMPERATURE Austin’s climate has already been warming and increases in annual and seasonal average temperatures are projected to continue for the foreseeable future. Hegewisch et al. (2019) and Brandt et al. (2020) have summarized observed trends in temperature in the Austin area as described below. • Temperatures have been increasing over the observational record in Austin, which goes back to 1938. From 1981-2010, average annual temperature in Austin was 52°F in the winter and 84°F in the summer, with an average minimum of 40°F and an average maximum of 98°F in the 30-year span. 42 • Maximum temperature has been increasing at a rate of 0.4°F per decade and mean and minimum temperatures have been increasing at a rate of 0.3°F per decade. Since 2000, all years have been above the 1961-1990 average. • Recent years have been increasingly hot. The decade 2000-2010 was the warmest on record for the contiguous United States and also for Austin. On average, about 10 days each year on which the heat index exceeded 105°F. • Six of the hottest 10-year periods in Austin have occurred between 2000 and 2019. These observed trends in temperature are projected to continue under all emissions scenarios. Both Jiang and Yang (2012) and Hayhoe (2014) predict higher temperatures than the present and similar rainfall as the present. Hayhoe predicts that near-term (until 2040) climatic changes in Austin will be similar under both low and high emissions scenarios. Likewise, Jiang and Yang predict that the surface temperature anomaly over Texas will be indistinguishable under the B1 (low), A1B (moderate), and A2 (high) emissions scenarios until about 2040 (Figure 6 from Jiang and Yang (2012)). Thus, any actions to reduce climate change are predicted to only affect temperature anomalies after 2040. By 2100, Hayhoe predicts 4.8°F and 10°F summer high temperature anomalies for the low and high emissions scenarios. Jiang and Yang predict 3.6-4.0°F, 5.4-5.8°F, and 6.1-6.5°F average summer temperature anomalies for the B1, A1B, and A2 emissions scenarios (calculated from Fig 9). Simulations under the three scenarios show more warming in summer than in winter. 5.1.3 PRECIPITATION Climate change effects on precipitation in the Austin region are less certain than effects on temperature (Brandt 2020). Slight decreases in annual precipitation are projected (Runkle et al. 2017), but the impacts vary by season and scenario according to different climate models. Hayhoe predicts lower annual rainfall totals (-2.1% and -6.8%) for low and high emissions scenarios. Hayhoe also predicts more dry days, longer dry spells, more days with intense rain events (precipitation > 2 in.), and more rainfall in the wettest five days of the year – in short, less or equal annual precipitation falling in fewer events. Jiang and Yang (2012) project slightly more (1.5%) annual rainfall in the B1 scenario and less (-3.0%) in the A2 scenario. They also predict more pronounced drying during winter (-2.7, -3.4, and -6.2% in the B1, A1B, and A2 scenarios). While periodic drought is a normal part of Texas climate, higher temperatures exacerbate the effects of drought, regardless of whether the frequency or intensity of drought changes. Even with unchanged precipitation patterns, higher temperatures are likely to intensify the effects of droughts that do occur (Young et al. 2017) (see Evaporative Demand, below). Although annual average precipitation may not change significantly, rainfall may be received in fewer and more extreme weather events (Hayhoe 2014). This may result in increased incidence of extreme flooding, as is being observed nationally. As a result, many municipalities are recognizing that FEMA’s 100-year mapping may underestimate risks, and municipalities are incorporating additional considerations including 500-year flood events into planning.1 For example, the City of Austin extends 1 “The term “100-year floodplain” is term used interchangeably with the “Special Flood Hazard Area” – the area that is subject to inundation from a flood having a 1-percent chance of being equaled or exceeded in any given year. The term “500-year floodplain” means that area which is 43 flood risk considerations beyond the FEMA floodplains by using a “fully developed” floodplain definition to guide permitting. The City also uses higher updated rainfall statistics to set design standards. The increased rainfall and potential increased frequency of extreme flooding has ecological outcomes as noted in Section 5.2. 5.1.4 EVAPORATIVE DEMAND The strongest climatic factor correlated with area of wildfire is vapor pressure deficit (evaporative demand) (Williams et al. 2013). Although projected precipitation changes are not statistically significant, and in and of themselves may not be meaningful for natural resources, the projected temperature changes are much more substantial and the confidence in those projects is much higher. Thus, although Austin’s rainfall patterns may not change substantially, temperatures during wet, normal, and dry periods will be higher. That will translate to increased drought stress and likely higher rates of mortality and wildfire that often accompany drought-stressed systems. Vapor pressure deficit (VPD) is the technical term for evaporative demand. Vapor pressure (VP) deficit = VPambient – VPsaturated. It is the difference between the ambient vapor pressure and the saturated vapor pressure at a given temperature. The greater the difference, the greater the evaporative “pull” on water bodies such as water inside a leaf. Even with hypothetically unchanged rainfall patterns (and assumed unchanged dew point temperatures), VPD will be exponentially higher due to higher temperatures alone. Higher temperature also reduces relative humidity (RH) which raises VPD linearly. So, even under a scenario of similar annual rainfall, higher temperature and the resulting reduced RH will compound to exponentially raise VPD and, correspondingly, evapotranspiration (McDowell and Allen 2015). Will et al. (2013) found, perhaps not surprisingly, that increased VPD due to higher temperature increased seedling mortality during drought conditions. Further, in the southwestern US, the warm season VPD is slightly better than the average maximum temperature at predicting forest drought stress (Williams et al. 2013). CLIMATE METRICS TERMINOLOGY Vapor pressure deficit (VPD) - The difference between the amount of moisture in the air and how much moisture the air can hold when it is saturated. It is used to estimate evaporative demand on plants and water bodies. Evapotranspiration (ET) - The processes by which water moves from the earth’s surface into the atmosphere. ET combines evaporation (the movement of water from the soil and water bodies) and transpiration (the movement of water vapor from within living plants) to the air. Precipitation-Potential ET (P-PET) – Rainfall minus potential evapotranspiration. Negative values represent a water deficit. subject to inundation from a flood having a 0.2-percent chance of being equaled or exceeded in any given year.” 42 U.S.C.§4101b(b)(1)(A)(i) and (ii) 44 Figure 5-1. Vapor pressure as a function of temperature. The red area indicates the temperature range in which we are operating with respect to climate change in Austin by 2100 (mean summer high temperatures increasing from 34C pre-2011 to almost 40C post-2070). In this range, the change in VPD as a function of temperature is steep. Table 4. Assuming no change in average summer dew point temperature (the authors’ assumption), by 2040, the average daily summer high vapor pressure deficit is projected to rise by 17.1%. By 2100, average daily summer high VPD is projected to rise by 27% and 60.1% under the low and high emission scenarios. Temperature anomalies are taken from (Hayhoe 2014). Average summer low RH is from 2017 weather data. 45 pre-20112011-20402041-20702071-21002041-20702071-2100emissions scenariolow and highlowlowhighhighsummer avg high temp (F)93.896.997.998.6100.2103.8summer avg low RH (%)40.937.236.0735.3133.6530.23dew point temp (F)66.466.466.466.466.466.4VPD3.1993.7453.9294.0634.3745.121change in VPD above pre-201117.1%22.8%27.0%36.7%60.1%Figure 5-2. Vapor pressure deficit under various temperature scenarios. As temperature increases, relative humidity (RH) decreases, assuming dew point remains constant. Thus, VPD increases slightly exponentially. Orange and red arrows indicate VPD at the low and high emissions scenarios, respectively. Temperature projections are taken from Hayhoe (2014). Average RH is from summer 2017. Under high emissions scenarios, VPD increases ~60% from 2011 to 2100. 5.1.5 AUSTIN’S TIMELINE FOR CHANGE Although climate change is already underway, potential ecological responses vary among species and habitat types. These in turn mediate system-wide responses to changes in temperature and precipitation. Schwantes et al. (2017) described the following climatic thresholds of drought mortality across Texas based on observations of the 2011 drought: • Warm season mean temperature anomaly +1.6°C • Vapor pressure deficit (VPD) anomaly +0.66 kPa2 • Annual precipitation deviation -38% from average • Precipitation - potential ET (P-PET) of -1,206 mm. Exceeding any of these thresholds corresponded with a substantial loss of tree canopy. Under high emissions scenarios, average temperatures are projected to exceed the +1.6°C threshold during 2040-2099, and VPD and P-PET conditions are projected to exceed their thresholds during 2070- 2099. Precipitation deviation is not expected to exceed -38% before 2100 (Schwantes et al. 2017). 2 KPa refers to kilopascal, the international unit equivalent to about 0.15 pound-force/inch. 46 Thus, based on above, the timeline for restoration to prepare natural areas for the changes in Austin’s climate in which scientists have high confidence is, conservatively, 2040. After 2040, the likelihood of crossing climate thresholds that will drive substantial changes in the structure and function of natural areas and increase the risk of negative outcomes such as intense wildfire and canopy mortality becomes increasingly certain. For management considerations, some of the above metrics may be more informative than others. For example, the temperature threshold was crossed during droughts of 1998 and 2011 in Austin, but the VPD threshold was crossed only during 2011. Widespread loss of trees was noted only in 2011. While this might be interpreted as tree mortality being more related to VPD than temperature, other factors also need to be considered such as climate conditions in the growing seasons prior to a drought. 5.2 ECOSYSTEM COMPONENTS 5.2.1 ABIOTIC COMPONENTS This section reviews physical components of ecosystems that affect the risk of negative climate-driven events such as canopy mortality and intense wildfire. Aspect Soil water availability varies on north-and south-facing slopes due to complex interactions between biotic and abiotic factors including both canopy and geologic structure (Murphy et al. 2020). South- facing slopes are associated with higher tree mortality in dry environments (Dorman et al. 2015) because solar radiation strikes more directly on southern slopes (in the northern hemisphere), increasing evapotranspiration and leading to drier conditions earlier in the growing season. For example, in Arizona, soil moisture on north-facing aspects remains higher during the spring and early summer compared with south-facing aspects (Murphy et al. 2020). Soil properties can be affected. In Idaho, soils on the north aspect retain as much as 25% more water at any given pore water pressure than samples from the south aspect slope (Geroy et al. 2011). Soil and geology As previously noted, soils vary at a broad scale from shallow and course in the Edwards Plateau to deep and fine-textured soils in the Blackland Prairie ecoregion. Soil water holding capacity is determined by soil texture, structure, and porosity in combination with landscape position as defined by slope, aspect, and topography. Water holding capacity affects drought-induced rates of mortality (Twidwell et al. 2014) as well as responses to management actions. For example, shrublands on coarse, sandy soils have been shown to rebound to pre-treatment conditions more quickly after brush removal and fire management than on fine clay soils (Wonkka et al. 2016). Tree mortality from drought has been found to be lowest in riparian and mesic sites in Texas (Schwantes et al. 2017). In a similar finding from California forests, tree mortality during and after drought is highest in the driest sites, likely because trees in dry areas are already close to their limits of climatic tolerance (Young et al. 2017). During the drought of 2011, about four out of five of the areas that experienced localized, but dramatic vegetation type-shifting tree mortality on the City of Austin Water Quality 47 Protection Lands were located in areas of especially shallow soil. Not all sites with shallow soil experienced widespread mortality, but of those that did, most were on the driest sites. By contrast, Twidwell et al. (2014) and Crouchet et al. (2019) each found higher mortality on the deeper soils of their study sites. On the sites surveyed by Crouchet et al. (2019), soil depth varied between 40cm (15.8in) and 150cm (59.1in), which are both well within the range of JUAS and QUFU rooting depth (Jackson et al. 1999). Twidwell et al. (2014) state that patterns of mortality are often context- specific (i.e. topoedaphic or geologic). They posit that on the Edwards Plateau, tree mortality during drought may be more directly related to the characteristics of the underlying geology. On their site, the deeper soils were underlain by a petrocalcic (cemented carbonate subsoil) layer that is relatively impermeable to both water and tree roots. Under normal conditions, trees on these soils have access to more water, and thus grow taller, but are more vulnerable during intense drought because of both tree height and lack of access to deep water sources. Shallower soils were underlain by fractured Edwards limestone, characterized by large fissures, holes, and pockets that allow the downward movement of soil, water, and tree roots. Under normal rainfall conditions, trees on these sites have access to less water and are shorter but are able to develop deeper root systems by exploiting soil-filled voids in the fractured bedrock. However, during drought conditions, these trees are less vulnerable to mortality because of their ability to access deeper water sources as well as lower photosynthetic demand related to their shorter stature. Overall, the breadth of research suggests that trees on dry sites are more vulnerable to mortality during drought. However, whether a site functions as dry or mesic may be affected by factors beyond soil depth. Other factors such as soil texture, soil organic matter, geologic characteristics, slope, and aspect may be equally or more important than soil depth in influencing tree mortality. Further, these factors, along with soil depth, may determine how and when sites shift from dry to mesic, both throughout the growing season and across wet and dry seasons. 5.2.2 BIOTIC FACTORS Species Characteristics Based on Darcy’s law (a principle hydrology modeling the flow of a liquid through a porous medium), McDowell and Allen (2015) identified the characteristics of trees that are most likely to die as a result of higher temperatures and drier conditions, which included trees that are: Isohydric (dramatically reduce photosynthesis during drought) 1. Taller 2. 3. Non-resprouting (are not able to generate new aboveground tissue after top-kill) 4. Coniferous 5. Have high leaf area (high leaf surface area per tree or per are of ground surface covered by canopy) 6. Low hydraulic conductance (low cross-sectional conducting area of stems per leaf area i.e. big canopy, small trunk) McDowell and Allen (2015) predict that these trees will be replaced by shorter, more xeric species. Shorter, more anisohydric species (continue to photosynthesize during periods of very low soil water availability) with relatively higher conductivity and/or higher stem conducting area per leaf area are 48 more likely to survive. In a global review, Greenwood et al. (2017) also found tree mortality from drought to be higher in trees with higher specific leaf area. With respect to woody plants common to the Austin area and the above list: • Number 1 likely indicates individual trees that are relatively tall for their species, rather than species whose individuals are taller on average. • Numbers 3-5 indicate Juniperus species such as Ashe juniper and eastern red cedar. • Number 2 may also indicate juniper. Isohydric plants are those that dramatically reduce photosynthetic activity in response to a drop in soil water availability. Anisohydric plants continue to photosynthesize, and therefore transpire, as soil water availability declines. These plants are able to tolerate very low leaf water potentials without experiencing cavitation but suffer increasing drought stress as soil water availability continues to decline. It is unclear if Ashe juniper is isohydric or anisohydric, or may be described by either term. Juniper exhibits behavior of both (Johnson et al. 2018). “Using a more recent definition of isohydry (hydroscape area) (Meinzer et al 2016), these species would be categorized differently with Ashe juniper and Texas persimmon (Diospyros texana) being more anisohydric…” Isohydry or anisohydry may not, however, affect vulnerability to all types of drought. Instead, each may confer benefits to either long-lasting, moderate drought or short-duration, intense drought as was experienced in 2011. Sade et al. (2012) demonstrate that anisohydric species are more productive under conditions of prolonged mild to moderate drought stress than isohydric species. Ashe juniper has been observed to exhibit relatively high mortality in intense drought. In the 2011 drought, mortality of Ashe juniper, Texas persimmon, live oak, and mesquite across the Edwards Plateau were 27%, 19%, 4%, and 0%, respectively (Figure 5-3) (Johnson et al. 2018). In Central Texas, Moore et al. (2016) found greater-than-expected mortality of Juniperus (mostly J. ashei) and lower-than-expected mortality of Quercus (mostly Q. fusiformis) and mesquite. On the Pruitt tract in eastern Travis County, Travis County land managers have witnessed broadleaf tree species such as black jack oak (Q. marilandica) and cedar elm (Ulmus crassifolia) released from competition following mortality of almost 100% of eastern red cedar (J. virginiana) that died during the drought of 2011. Schwantes et al. (2017) found that one of the ten ecological systems most impacted by the 2011 drought was what they term ”Ashe juniper motte and woodland” on the Edwards Plateau. In contrast, they found that three of the ten least impacted ecological systems were “shin oak shrubland,” “deciduous semi-arid shrubland,” and “deciduous semi-arid slope shrubland,” also on the Edwards Plateau. 49 Figure 5-3 Tree mortality for four common Texas woody taxa – Juniperus (juniper species), Diospyros (Texas persimmon), Quercus (oak species), and Prosopis (mesquite) following the drought of record in 2011. From Johnson et al. 2018 Community Characteristics Community characteristics such as stand age and density also affect risk. To examine the effect of community factors in drought effects, Crouchet et al. (2019) asked if crown mortality was consistent across species four years after the 2011 drought. They found that the factors explaining mortality for Ashe juniper and live oak were related to competition and plant community structure. Large Ashe junipers were less likely to die, Ashe juniper were more likely to die where the stem area density was high, and trees at higher elevations were more likely to survive. This was surprising, since thicker soil is usually associated with more favorable growing conditions and lower elevations. The researchers attributed this finding to the trees’ abilities to access water in fractured bedrock below the soil layer, which is closer to the surface in shallow soils. Larger live oaks were also more likely to die, but oak trees in areas with higher total stem density were less likely to die. Texas persimmon died more often in places where there were more days that the vapor pressure deficit reached 55 kPa and more often on south-facing slopes (Crouchet et al 2019). The northward migration of climatic zones has the potential to facilitate the concomitant northward migration of invasive species that currently occur to the south or west of the Austin area (Bradley et al. 2010). Such invasive species include buffelgrass (Cenchrus ciliaris), tanglehead (Heteropogon contortus), cheatgrass (Bromus tectorum), bahiagrass (Paspalum notatum), guineagrass (Megathyrsus maximus), and salt cedar (Tamarix sp.). Indeed, Austin has begun to witness the movement of other invasive species such as silky bluestem (Dichanthium sericeum) and Kleberg bluestem (Dichanthium annulatum) into the Austin area since about 2005-2010. Plant diversity Biodiversity maintains ecosystem function after disturbance because more species provide greater guarantees that some will maintain function even as others fail (Yachi and Laureau 1999) through a 50 variety of mechanisms. In forests for example, a global study (Anderegg, et al., 2018) found that diversity of hydraulic traits was the best indicator of drought resistance – specifically the “hydraulic safety margins, the difference between Psimin and P50“ (which is the water potential at which 50% of stem xylem conductivity is lost). In a review of 46 experiments in grasslands, Isbell, et al. (2015) tested the role of biodiversity in response to climate and found more diverse communities recovered faster under a broad range of climate conditions. However, they note that “by a year after each climate event, ecosystem productivity had often fully recovered, or overshot, normal levels of productivity in both high- and low-diversity communities, leading to no detectable dependence of ecosystem resilience on biodiversity.” Therefore, it may be the increased rate of recovery offered by diverse grassland communities is the important factor for resisting invasive species encroachment in climate stressed systems. In Texas grasslands, Xu et al (2017) examined the recovery of more diverse versus more homogenous communities after the 2011 drought event. Although their work included non-native species that could be confounding, they found that C3 forbs were resilient to drought in native communities and often increased in relative abundance during the studied recovery period, especially in diverse mixtures. More generally, drought changed community composition toward greater forb and short grass abundance that continued following drought relief in both native and exotic communities. Tree density Across multiple systems, high tree density tends to correlate with higher drought mortality (e.g. Bottero et al. 2017). Young et al. (2017) state that during drought, “Higher levels of competition within a forest stand can increase mortality probability (Das 2011, Dietze and Moorcroft 2011, Ruiz-Benito 2013, Zhang 2015, Van Mantgen 2016).” Further, “It is widely appreciated in forest management that lower density improves forest resistance and resilience to drought (Bottero 2017)” (Crouchet et al. 2019). In California, forest stands with high live basal area experienced higher-than-expected mortality during drought (Young et al. 2017). The relationship between tree density and mortality was weak, however. Further, the relationship between live basal area and mortality was stronger on drier sites and in drier years (Young et al. 2017). Crouchet et al. (2019) found that on the Edwards Plateau, J. ashei mortality increased with basal area of both juniper and D. texana. However, Crouchet et al. (2019) also found that Quercus fusiformis mortality was negatively related to total species density. Canopy height & structure The physical structure of forests affects their response to drought events, with greater tree height a general predictor of greater mortality risk (Stovall et al. 2019). Across Texas, Moore et al. (2016) found that within species, the 2011 drought affected larger tress more than smaller trees (dead trees were 17- 20% larger than live trees). This effect was more pronounced in mesic areas (Moore et al. 2016). That is, in mesic areas, dead trees were much larger than average whereas in dry areas, dead trees were only slightly larger than average. Polley et al. (2016) also found that Ashe juniper trees that died in 2011 were, on average, larger (by canopy area) and had been growing more slowly than average for the decade preceding 2011. At the Sonora research station, Twidwell et al. (2014) found that taller species, such as juniper and live oak, were more vulnerable to mortality than understory shrubs during the 2011 drought. Juniper had higher mortality in the overstory than understory (Twidwell et al. 2014). This pattern was observed in the droughts of both 2011 and the 1950’s (Twidwell et al. 2014). For Ashe juniper trees in central Texas savannas, Polley et al. (2018) found that mortality risk was highest among large trees. We have also observed this on the WPQL. Rapid recruitment of competition-released 51 juvenile JUAS after drought-kill of larger, “parent,” trees results in high density of smaller Ashe juniper in years following drought. In contrast to these studies, Crouchet et al. (2019) found that for Ashe juniper and live oak (Quercus fusiformis), small trees (low basal area) died at higher rates than large trees. 5.3 CLIMATE ANALOGUES Climate analogues are vegetative communities that currently exist in what are projected to be the future climatic conditions of another area. Fitzpatrick and Dunn (2019) report that the current climate of Nuevo Laredo, Mexico, is most like Austin’s projected climate in the year 2080 under both optimistic and unmitigated emissions scenarios (RPCs 4.5 and 8.5, respectively). Figure 5-4. Climate similarity maps Climate similarity maps for Austin in 2080 under unmitigated (RCP8.5) and more optimistic (RCP4.5) emissions scenarios, respectively. Lines (red in A, blue in B) connect Austin to the city (Nuevo Laredo, Mexico) with the highest similarity to Austin’s projected 2080 climate in each scenario. https://fitzlab.shinyapps.io/cityapp/. Beck et al. (2018) project that under RCP8.5 (unmitigated emissions), climate classifications in central Texas will migrate eastward by late century. Austin’s proximity to the BSh (hot arid steppe) system will increase as the BSh zone expands to the north and east, which could result in vegetative shifts especially on exposed sites, particularly in western Travis County. The western edge of the range of Ashe juniper in the Edwards Plateau currently corresponds well to the Cfa/BSh (temperate, no dry season, hot summer)/hot arid steppe) boundary. The modeled eastward shift of the boundary is a response to hotter and drier conditions that encourage vegetation dominated by grasses and shrubs. Though juniper-oak woodland may remain dominant in the eastern Edwards Plateau, it is likely to be under significantly more environmental stress than at present, perhaps declining in more xeric areas, such as on south and west-facing aspects, convex (drier) hill slopes, shallow soils, and low elevations. 52 Figure 5-5a. Current climate classifications in central Texas. From Beck et al. (2018). 53 Figure 5-6b. Projected climate classifications in central Texas under RCP8.5 (unmitigated emissions). From Beck et al. (2018). Note the overall eastward retraction of the Cfa classification and the extension of the BSh classification down the Colorado River valley. City of Austin PARD staff mapped the locations in North America where Austin’s projected temperature and rainfall currently occur under low, moderate, and high emissions scenarios. The nearest mean annual temperature and rainfall analogue for the low emissions scenario is between Corpus Christi, TX and Beeville, TX. For the moderate and high emissions scenarios, the nearest analogues are at higher elevations between Monterrey and Tampico, MX (near San Nicolás, San Carlos, and Las Minas). 54 Figure 5-7. COA-created temperature and rainfall analogue. Locations in North America where Austin’s projected 2070-2099 summer temperature and rainfall currently occur under low, moderate, and high emissions scenarios (based on Jiang and Yang, 2012). Temperature is project to increase significantly. Average annual precipitation change is anticipated to be negligible, but more variable – fewer, more intense rain events and longer dry periods. Analogues for summer (Jun-Aug) conditions may be more informative than analogues for annual conditions because summer is when environmental stressors on woody species are most intense. The nearest summer temperature analogues for low and moderate emission scenarios are Nuevo Laredo, Texas and Salinas, Mexico, respectively, in the Rio Grande valley. For high emissions scenarios, temperature analogues are in and around Phoenix, AZ. All temperature analogues, however, are currently drier than projected rainfall analogues; therefore, there are no suitable analogue locations representing both temperature and precipitation projections simultaneously. That is, there are no large areas in North America that currently experience Austin’s projected summer temperature and rainfall under climate change scenarios. Suitable temperature and rainfall analogues may exist elsewhere in the world in very hot areas that receive moderate annual rainfall, but staff were not able to acquire sufficient geospatial data to address that question. 55 5.3.1 INTERPRETING CLIMATE ANALOGUES While climate analogues can be useful for visualizing and predicting long-term climate, hydrologic, and habitat changes that may result from climate change, there are at least two weaknesses of climate analogues. The first is illustrated with the COA-generated maps based on Jiang and Yang (2012), i.e., when temperature and precipitation projections do not share a geographic location. This lack of a reference can be challenging for climate change planning. Second, the vegetative habitat of any area is influenced by non-climate factors such as topography, aspect, soil type, and geology that are not accounted for in the climate analogue models. This complicates the application of climate analogues that link areas with dramatically different abiotic conditions. We see this in the climate analogues for the eastern Edwards Plateau. Perhaps the most dominant topoedaphic element of this ecoregion is karst geology. However, the Fitzpatrick analogue (Nuevo Laredo) and COA temperature analogues (Rio Grande valley) have different geology and soil types. Ashe juniper, one of the most abundant tree species in the eastern Edwards, appears to be geographically constrained not by temperature or precipitation, but by geology and soil type, or at least land uses that are constrained by geology, topography, and soil type. Thus, whether Ashe juniper populations accompany a climate shift to the Rio Grande Valley remain, as does whether the species would be extirpated from the Edwards Plateau because of climate stress, or would it persist. An examination of the topoedaphic and climatic conditions of disjunct areas of the Ashe juniper range in Mexico would be helpful in answering these questions. In the end, climate analogues may be most useful in helping us understand the ways in which plant communities are likely to change and where those changes may be the most pronounced. The analogues and projections above indicate that we are moving toward a climate that is associated with dry woodland, grassland, and/or scrub. Given certain topoedaphic conditions and land uses, we should expect current vegetation communities to change accordingly. We should also expect this change to be accompanied by significant plant mortality of mesic species and on xeric sites. 5.4 CLIMATE-DRIVEN NATURAL AREA OUTCOMES The predicted changes in temperature and precipitation will affect native plant communities in a variety of ways. Among these, the most important climate-driven outcomes for the vegetative communities in Austin’s parkland natural areas are changes to canopy mortality rates, community composition, woody encroachment into grassland, altered recruitment patterns, intense wildfire, and novel flood dynamics. It is worth noting some limitations in our ability to predict changes in plant community response to climate change. The 2011 drought, noted above, was the driest year on record for Texas and has given rise to a rich body of scientific literature that helps us predict the outcomes of future droughts. However, it is important to consider that this research is based on discrete events, so while it helps establish the mechanisms of change it does not address how and how fast they play out for vegetation if high VPD is a protracted or permanent condition. In the future, drought is likely to occur more frequently and for longer periods. This is an important caveat to this review of the current research and predictions. 56 5.4.1 CANOPY MORTALITY Higher temperatures typically increase rates of evapotranspiration (Choat et al. 2018). Combined with drought, these stresses can lead to dieback and mortality. As described previously, abiotic factors such as geology and soils, as well as species characteristics, influence the susceptibility of sites to canopy mortality. 5.4.2 COMMUNITY COMPOSITION Climate change will extend Austin’s growing season, and Austin’s USDA Plant Hardiness Zone will shift from 8 to 9 by 2100. Plant Hardiness Zones predict which species can survive the winter, and shifting zones imply potential for new plants to move in from warmer southern regions. Increases in temperature paired with extended growing seasons will likely benefit non-native invasive species, particularly following die-back events (Brandt 2020). Another factor to be considered is the photosynthetic pathway. Most trees and shrubs utilize the C3 pathway, which will is less efficient under higher temperatures (Nordt et al. 1994). On the other hand, C3 photosynthesis is more efficient with higher CO2 concentrations. Possible scenarios include (1) lower C3 efficiencies from higher temperatures – combined with higher ET, potentially longer droughts, and more fire disturbance – leading to more open, herbaceous- or shrub-dominated systems; (2) higher C3 efficiency from CO2 enrichment – combined with reduced opportunities for prescribed fire and possibly wetter summers (Hayhoe 2014) – encourages shrub encroachment into grasslands (Archer et al. 2017); and/or (3) woodlands and grasslands converge toward shrubland. 5.4.3 REDUCED RECRUITMENT Seedling and sapling mortality is a consequence of severe drought (Hanson and Weltzin, 2000). For many Texas hardwood species, recruitment is poor in many areas, though both fire management and deer control can recruitment increase rates (Andruk et al. 2014). For most woody species, recruitment is episodic, associated with high rainfall or high rainfall coinciding with disturbances such as fire. As conditions that facilitate recruitment become less frequent and drought and extreme heat events become more frequent, recruitment dynamics will be altered. For many mesic species, recruitment may fall below baseline mortality. Even species whose mature individuals are able to persist through more extreme droughts may not be able to recruit new individuals. These species will certainly decline over time in all but the most accommodating microclimates. 5.4.4 INTENSE WILDFIRE Climate change will increase the likelihood of crown fire by increasing drought stress. When climate alone in considered, higher temperatures with equal or less rainfall should result in higher evaporative demand, which, coupled with longer dry periods, would be expected to result in drier wildland fuels and increase the frequency and intensity of wildfire (Stambaugh et al. 2018). Live fuel moisture is the proportion of the weight of living plant material comprised by water. The live fuel moisture of Ashe juniper is used by fire management professionals in the Austin area as a key indicator of crown fire potential. Low live fuel moisture indicates a high potential for crown fire involvement. During summer months, low live fuel moisture is tightly correlated with the Keetch-Byram 57 Drought Index (McCaw et al 2018) which reflects soil water availability. Thus, longer growing seasons with higher temperatures are likely to result in longer periods of low soil water availability which is likely to increase the both the potential for crown fire activity during dry times as well as the length of time that crown fire potential is high. As development in the Austin area continues, we would also expect an interaction between vegetation and WUI expansion to affect wildfire frequency and intensity. Most wildfires in central Texas are human-caused and start in the WUI (CWPP). Thus, WUI expansion would be expected to increase the frequency of ignitions (Liu et al. 2015, Radeloff et al. 2018)]. Similarly, WUI expansion makes maintenance of natural areas with prescribed fire more difficult due to the increase and proximity of smoke receptors such as homes, businesses, and roads. Therefore, WUI expansion would be expected to facilitate an overall increase in canopy cover or fuel loading. Taken together, an increase in woodland cover and fuel loading coupled with an increase in wildfire ignitions in a hotter climate would be expected to increase the frequency of intense wildfires or the intensity of wildfires that do occur. 5.4.5 NOVEL FLOOD DYNAMICS “Flood risk” can be considered somewhat of a misnomer in the context of management of natural areas. High seasonal flows that overtop streambanks are natural events and are needed to create and maintain functional riparian habitats and vegetative communities. However, given land use changes that increase impervious surface (resulting in abnormally high stormflows with novel pollutant loads into waterways) and under conditions of increased storm intensity, there will be some climate-driven outcomes related to stormflows that have ecologically meaningful effects. Stream channels in developing watersheds are especially vulnerable to increased rates of erosion (down-cutting and widening). Floods that reach higher into upland areas of may also facilitate colonization of invasive species. In many systems, drought increases sediment in waterways (Dunbar 2010), as dry soils are more likely to erode. Systems that are freshly scoured out by flooding are also prone to colonization by invasive species. 5.5 SUMMARY OF CLIMATE VULNERABILITY LITERATURE In summary, a thorough review of the scientific literature indicates changes, potentially subtle to dramatic, to natural area form and function. We expect changes in Austin’s climate to continue imposing pressure in the direction of compositional shifts toward short-statured, more xeric vegetative communities resulting from increased baseline tree mortality rates or discrete mortality events. There is also evidence in the literature as well as case studies from across the region of both grasslands and woodlands tending toward shrubland. As with many other temperate regions across the world, fire seasons are expected to lengthen and wildfire frequency, size, and intensity are expected to increase on balance in response to a variety of anthropogenic, biotic, and abiotic factors. It is likely that these changes will be driven by many factors pertaining to climate, abiotic site characteristics, the vegetative community, and species composition. First, climatic characteristics such as high temperature and vapor pressure deficit (evaporative demand) will increase soil drying and plant water stress. All things equal, the most dramatic changes are expected on the most resource-limited sites, identified by characteristics such as shallow or course soils with low soil water availability as well as potentially steep, south- or west-facing slopes. However, plant community characteristics that 58 determine resource competition will play a significant role in driving vegetative change and climate risk as well. Such elements include tree stem density and/or basal area, canopy bulk density, vegetative structure (i.e. fuel model), and fuel loading. With respect to species composition, certain species or life strategies have been and are expected to continue to be disproportionately vulnerable to increased temperatures and/or resource limitation. Such characteristics include woody species that are mesic (require moderate to high water or nutrient availability), non-resprouting (do not resprout after top-kill), coniferous, as well as individual trees, regardless of species, that are tall and have a high leaf area high leaf area per conducting area (full crown with a small trunk). The above narrative describes the dynamics that are high-confidence and expected to influence central Texas natural areas consistently throughout the 21st century. However, there is a certain amount of increased climatic variability that is also expected. This variability will be expressed as a variety of unpredictable, anomalous, discrete events including floods, winter storms, ice storms, hail, damaging wind events, and short but highly intense drought. Such events are all normal occurrences in the Austin area, but their intensity is expected, at times, to be more extreme. Fortunately, the strategies for preparing for these somewhat anomalous events mirror those for addressing the high confidence factors of extreme heat, drought, and wildfire. In addition to improving the resilience of natural areas to climate risks, preparing for the types of discrete, anomalous events mentioned above will require building and maintaining organizational capacity and resilience as well. This will involve building work units that have the knowledge, skills, resources, and discretion to quickly adapt, reorganize, and mobilize to address challenges in the moment. Natural resources work units within other agencies in the Austin area have been deployed in recent years to help support response to wildfire, storm damage, floods, and even search and rescue. Fortunately, ecological restoration at large scales requires the types of versatile knowledge, skills, and resources that are transferable to a variety of needs. Thus, building the high-reliability organization needed to implement the recommendations in this land management plan will do much to prepare PARD for responding to the kinds of anomalous events mentioned above. 5.6 CLIMATE SMART STRATEGIES Climate smart strategies use existing management practices with the added focus on how location, timing, and intensity of actions can be adjusted in the face of increasing climate stresses. There are several traits that make natural systems better able to recover from stresses and shocks such as extreme heat, drought, wildfire, flood, disease, and invasive species. In each site and system, land managers can work to improve resilience by supporting or enhancing characteristics such as listed below. ● Structure o Structural components of ecosystems that can affect resilience include herbaceous cover, canopy cover, tree density or basal area, canopy bulk density, fuel loading, and fuel arrangement. As discussed further in the geospatial portion of the vulnerability analysis (Section 5.7) and in Section 6, the structure of vegetative communities can improve or constrain resource availability and thus determine the likelihood of negative 59 outcomes when resources become scarce, such as during a drought or extreme heat event. ● Composition o Community composition can affect resilience. Inherently, vegetative communities with a high proportion of species that are adapted to projected future conditions will themselves be more productive, stable, and resilient to those future conditions. For example, a highly diverse mesic woodland may not necessarily be resilient to future conditions that are dramatically hotter or drier than at present. Thus, it is critical given the current rate and trajectory of climate change to manage systems toward states that will be resilient in future conditions. ● Diversity o Diversity is the product of the number of different elements in a system and their arrangement or distribution. Thus, ecological diversity is the product of both the number of species in a system (called “species richness”) as well as their demographic distribution. Ecological diversity may also refer to the degree of genetic variation, the number of distinct vegetative communities across a site, and/or the structural complexity (e.g., the number of vegetative layers). o Highly diverse systems tend to support higher levels of vegetative productivity and generally recover from disturbance more quickly than less diverse systems. Maintenance of function and rapid recovery and maintenance of function are facilitated by complementarity (many species with different niches) as well as redundancy (many species with similar niches). o Diverse hydrologic regimes and soil types can also facilitate adaptive capacity. Over long time scales, the many complementary and redundant pieces of diverse systems enable recombination and adaptation. o Physical connectivity of natural systems affects species’ ability to move across a landscape. Connectivity enhances resilience by enabling species to continue critical life processes and disperse their populations, especially in response to disturbance. Natural areas in urban landscapes are usually highly fragmented by manmade barriers that species often cannot cross. Restoring corridors to connect larger habitat patches can be an important strategy for improving resilience. ● Connectivity 5.7 GEOSPATIAL ANALYSIS As described in the preceding subsections, climate trends in Austin are expected to result in multiple added stresses to native plant communities including increased tree mortality, increased invasive species, shifts in plant community composition, and increased likelihood of wildfires. The risk of drought and other climate-driven stressors responds to local, site-scale conditions such as topography, aspect, and soil moisture (Schwantes et al. 2018). Therefore, the geospatial analysis assesses the vulnerability to Austin’s parks at both the system-wide scale and at the individual park scale. 5.7.1 PRELIMINARY SYSTEM-WIDE CLIMATE HAZARDS 60 A preliminary evaluation used available data for individual hazards to understand the locations and extent of threats to focal areas in Austin Parks from wildfire, extreme heat, drought, and flooding. Initial data sources and limitations are reviewed below. Wildfire Two data sources from the Austin Fire Department Wildfire Division were evaluated for potential inclusion in the vulnerability assessment. • The Travis County Wildlife Hazards map assesses the relative potential for exposure of structures to wildfire as well as the locations of the greatest potential cumulative structure damage. The community wildfire risk algorithm includes slope, flame length, rate of spread, structure density, and proximity of structures to wildland fuels. Flame length and rate of spread are outputs from FlamMap modeling based on fuel type. The fuel type data that underlie the Travis County Wildfire Hazards map was incorporated into the field assessment and climate vulnerability analysis portions of this document. However, the Travis County Wildfire Hazards map outputs had limited utility for the park vulnerability assessment due to incorporation of structure density and proximity, which elevate the modelled risk in areas with high structure density in close proximity to wildland fuels. The Travis County Wildlife Hazards outputs are currently and may continue to be used by PARD as an assessment tool for project planning, depending on the intent and context. • Crown fire probability. FlamMap outputs fire behavior characteristics based on slope, fuel model, tree canopy characteristics, fuel moisture, and simulated scenarios for various weather parameters. Model outputs include eight parameters, two of which (crown fire activity and burn probability) were found in combination to be most relevant for assessing relative fire risk to focal areas in Austin Parks. Figures 5-8 and 5-9, show the system-wide and example site-scale crown fire probability. 61 Figure 5-8. Crown fire probability on PARD natural areas within study area. 62 Figure 5-9. Crown fire probability at Trevino Metro Park. 63 Extreme Heat Two information sources were reviewed for potential inclusion in the current study. • Trust for Public Lands (TPL) heat island data o TPL created an index as part of the Healthy Parks Plan for Travis, Bastrop, and Caldwell Counties (Austin Parks Foundation 2019) with a focus on identifying potential locations to mitigate urban heat island. The index combined information on heat islands, poor air quality, and lack of tree canopy, which was found to have limited utility for assessing potential influences of localized heat island in parkland natural areas. o The dataset references the same type of Landsat data as in the NASA DEVELOP study below, therefore directly using NASA data was found to be more applicable. • NASA Landsat Land Surface Temp via NASA DEVELOP o Daytime temperatures from April to September for the years 2015-2020. o Analysis was based on standard deviations from the mean daily summer temperature for all parks in the study. o The parklands in the study area reflected the general pattern of higher surface heat areas associated with impervious surfaces, structures, and other development around and within parks and their influence beyond their immediate footprint. Figure 5-10 shows the distribution of heat intensity within parklands across the study area. 64 Figure 5-10. Extreme heat in PARD lands within study area. Drought To assess drivers of drought and available geospatial data, a desktop analysis of standing dead tree areas was conducted. The standing dead tree area data was sourced from available fire modeling FlamMap inputs and field observations. In review of aerial photographs from previous years, the appearance of the standing dead tree areas was evident following extreme drought (i.e., post 2011), leading to the assumption that drought was a major contributor to the documented tree mortality. The standing dead tree area locations were tested for correlations with slope, aspect, elevation, clay percentage, soil erodibility, soil depth, and soil water availability. Some correlation was found with soil depth, and even stronger correlation with soil water availability, which also incorporates soil depth. A correlation was not found with the other variables tested of slope, aspect, elevation, and clay percentage. This analysis 65 supported the use of soil water availability as a key indicator for drought hazard, with low water availability indicating high drought hazard. Woodland located in the “highest” and “high” hazard categories of soil water availability has over 20 times more standing dead tree area than woodland located on the “lowest” and “low” hazard categories. These categories should be thought of spatial gradients of hazard in which numerous site-specific factors, as described above, will also combine to influence outcomes. Figure 5-11 shows the system-wide distribution of soil water availability, and 5-12 is an example for Trevino Park of a zoomed in view. Figure 5-11. Soil water availability in PARD lands within study area. 66 Figure 5-12. Soil water availability in Trevino Metro Park. 67 Flooding Floodplain data were reviewed to identify bottomland areas which may be supported by routine flooding as well as areas that may be exposed to increased intensity of erosion from flooding events. Datasets included: • The 500-Year (0.2-percent chance of being equaled or exceeded in any given year) FEMA floodplain is shown in Figure 5-13, which is currently used as Austin’s regulatory 100 Yr floodplain. (An interim regulatory step until modeling is updated, which will take a couple of years). • The Austin Fully Developed 100-yr floodplain, which is the current Austin regulatory 25 yr floodplain. Note, this is because the City of Austin Watershed Protection Department regulates development in Austin based on the fully developed condition floodplains rather than the FEMA floodplains, which represent existing conditions in the watersheds. Fully developed means that we consider land cover (land use and impervious cover) based on the maximum allowable impervious cover based on zoning or based on watershed or other limits outside of the City’s zoning jurisdiction. In some of the more heavily urbanized watersheds near the core of the city, there is essentially no difference between existing and fully developed condition land use. In suburban and rural areas, using the 25- yr mapping makes a bigger difference for planning. 68 Figure 5-13 Flood mapping for PARD lands within study area. Preliminary Hazard Mapping Application As part of the current project, geospatial analyses were explored to test creating composite indices and linking data to vegetation communicates and site conditions. While an initial all-in-one index was not useful for planning, initial overlays using indicators listed below were helpful for guiding the field assessment of site conditions described in 5.7.2. 69 Table 5. Hazard indicators Indicator 1 Lowest Vulnerability 2 3 4 5 Highest Vulnerability Wildfire Risk Low Moderate Elevated High Highest Heat Intensity Soil Water Availability <-1/2 SD >1/2 SD Highest Soil Water Availability -1/2 SD to Mean Mean to 1/2 SD 1/2 SD to 1 SD >Mean to 1/2 SD -1/2 SD to Mean <-1 SD to - 1/2 SD >1 SD <-1 SD Lowest Soil Water Availability Fully Developed Austin 100- year (New Regulatory 25-year) Flooding Outside 500- year - Resilience and Connectedness Most Resilient, More Resilient Slightly More Resilient Average/Median Resilient Slightly Less Resilient Less Resilient, Least Resilient, Developed 500-year FEMA (New Austin regulatory 100-year) Data Notes Composed of Slope, Structure Density, Flamelength, Rate of Spread, and WUI Proximity inputs. Daytime Land Surface Temp Available Water Storage (AWS) 0 to 100cm With future updates by Watershed Protection Dept. to Atlas 14 Data, flood frequencies will shift. (100-yr becomes ~25-yr, etc.) Composed of Local Connectedness and Landscape Diversity Data Source Austin Fire Department Wildfire Division Landsat via NASA Develop NRCS SSURGO City of Austin Watershed Protection Department TNC Resilient Sites 70 5.7.2 CONDITION ASSESSMENT INPUTS In addition to remote data inputs, an on-the-ground rapid assessment was conducted to support the vulnerability analysis. The rapid assessment is intended to give an indication of overall system-wide condition with high-level characterizations of polygons. Detailed assessments of each site should be conducted to confirm and update the assessment over time. The preliminary geospatial analysis was used to designate focal areas to help guide site visits. Areas with high hazard values and areas of potentially high resilience or potential for species diversity enhancement were prioritized. Areas with high resilience potential were identified using a combination of the TNC Resilient Sites, ecological sites, hydrology, and conversations with local ecologists familiar with the area. Communities were assigned condition scores based on the following scoring framework and characteristics which indicate ecological condition, the potential challenges facing them and their adaptive capacity. Condition Scoring A subset of data gathered was used to assign condition scores to the points. Appendix 1 describes the basis for assigning numerical scores, with higher numbers representing better condition. The scores were then broken into 5 categories using the Natural Breaks function within GIS and reclassed on a 5 point scale to indicate condition. Point scores were used to create community condition polygons. • 1 - Very poor • 2 - Poor • 3 - Moderate • 4 - Good • 5 - Very good A simplified version of the algorithm used to develop the scores is given below. Condition= structural diversity + compositional diversity + soil condition – invasive cover – potential impacts + adaptive capacity. A general score was calculated for all communities and then parameters specific to community type were added in a community specific score. Condition = General score (all communities) + Community specific store (bottomland/riparian, woodland, grassland) Community specific scores are: ● Grassland = General Score + % cover decreaser grass + forb cover + Microtopography ● Woodland = General Score - Fire risk - fuel condition + # of layers present + woody age diversity ● Bottomland/riparian = General Score + Fuel condition + Stabilizer species present + Structural diversity + riparian buffer + floodplain connectivity Results of the condition assessment are shown in Figure 5-14, individual parameters are outlined in the following section, and further in Sections 6 and Appendix 2. 71 Figure 5-14. Plant community condition for management areas based on 2022 rapid assessments. 72 Characteristics for All Plant Communities Eleven parameters of condition were assessed for all plant communities as listed below. Parameters that were assigned cover classes used the categories shown in the table below. All cover estimates were based on visual percent cover estimates for an area with an approximate 30m radius, centered on a GPS’d point. Parameters other than cover, which were assigned scores for high/low classes used a scale of 1: very low, 3: moderate and 5: very high. ● % cover of each layer (overstory, midstory, ground layer) 1-5 ● % cover litter 1-5 ● % cover bare ground 1-5 ● % cover Invasive species ● Species richness by layer, and overall community 1-5 ● Locally rare. i.e., uncommon species presence/absence ● Soil conditions described in Table 5 ● Erosion severity 1-5 ● Erosion extent – Cover class 1-5 Overall condition scores are described further in the Methods appendix. Table 6. Soil Condition Classes % Cover classes Class 1: 0- trace Class 2: 1-25% Class 3: 26-50% Class 4: 51-75% Class 5: 76-100% Characteristic Class 1 Class 2 Class 3 Class 4 Class 5 Soil and debris deposited against minor obstructions Moderate movement of soil particles has occurred Some movement of soil particles has occurred No visual evidence of soil movement Soil movement Surface rock and/or litter Subsoil exposed on much of the area; may have embryonic dunes an/or wind scoured depressions Very little remaining; if present, surface rock or fragments exhibit some movement and accumulation of smaller fragments behind obstacles 73 Extreme movement; many large deposits against obstacles; surface rocks exhibit movement; smaller fragments accumulate behind obstacles Moderate movement; fragments deposited against obstacles, fragments have a poorly developed distribution pattern May show slight movement; if present, coarse fragments have truncated appearance or spotty distribution caused by wind or water Accumulation in place; if present, the distribution of fragments shows no movement caused by wind or water Pedestaling Most rocks and plants pedestaled and roots are exposed Many rocks and plants pedestaled and roots are exposed Rocks and plants pedestaled in flow patterns Slight pedestalling in flow patterns No visual evidence of pedestaling Characteristic Class 1 Class 2 Class 3 Class 4 Class 5 Flow patterns Rills and gullies Flow patterns numerous, readily noticeable; may have large barren fan deposits May be present at depths of 8--15 cm and at intervals of less than 13 cm; sharply incised gullies cover most of the area, with 50% actively eroding Flow patterns contain silt, sand deposits and alluvial fans Well defined, small and few with intermittent deposits Deposition of particles may be in evidence No visual evidence of flow patterns Rills 1-15 cm deep at 150 cm intervals; gullies numerous and well developed; active erosion on 10-50% of their lengths or a few well- developed gullies with active erosion along more than 50% of their length Rills 1-15 cm deep in exposed places at about 300 cm intervals; gullies well developed, with active erosion along less than 10% of their length with vegetation present Few infrequent rills in evidence at distances of over 300 cm; evidence of gullies with little bed or slope erosion; some vegetation is present on slopes No visual evidence of rills; may be present in stable condition, but with vegetation on channel bed and side slopes Characteristics for Specific Plant Communities Community characteristics specific to general community type (grassland, woodland, bottomland/riparian) are below: • % cover decreaser grasses • % cover forbs Grassland Woodland • Structural layers present (1-3, related to number of strata- overstory, midstory, ground layer) • Woody age diversity 1(single class) – 5 (all ages present). Class 1 – the woodland contains trees that are primarily a single age and relatively uniform in size. Class 5 – the woodland contains different sizes and ages, with fairly even distribution across the classes. The classes are seedlings, saplings, mature trees. • Density. Density was estimated in two ways – assignment of a fuel model (Timber, shrub, grass) and prism estimation of basal area. The latter proved challenging in very dense understory conditions and the results were not used in further analysis. 74 Bottomland/riparian • Stabilizer species present/absent (Nelle 2009) • Structural diversity present/absent. Within riparian communities, structural diversity refers to the presence of both herbaceous and woody species, not to strata • Riparian buffer of at least 25’ with present/absent • Stream channel connected to floodplain yes/no Potential threats • Indicators of potential wildfire risk: ○ Fuel model group (timber, shrub, grass), ○ Fuel characteristics Fuel loading, tree density and arrangement were combined into a high/low score. High fuel loading +high tree density + presence of ladder fuel=high. • Flood: evidence of flood damage to soils or vegetation, connectivity of stream channel to floodplain, presence/width of riparian buffer, structural and species diversity of bottomland/riparian community • Heat: Vegetation mortality Adaptive Capacity • Cover of adaptive grasses/trees. Adaptive trees were defined as species receiving low vulnerability scores in the Vulnerability Assessment of Austin’s Urban Forest and Natural Areas (Brandt et al. 2020). Adaptive grasses were defined as climax perennial species identified in the reference community for ecological site. These species have characteristics suggesting adaptability to expected conditions (Anderson et al. 2018, Swanston et al. 2016). • Microtopography/mesic areas presence/absence • Pollinator plant cover • Connectivity • Seed source for dispersal (i.e., for future harvest opportunities) • Management access is defined as access for personnel and equipment for potential management activities, with an emphasis on access routes and an ability to access the area with vehicles and equipment. Past Management Past management practices exert a strong influence on current conditions. Past management can be inferred based on condition. For example, a grassland dominated by increaser species and missing decreasers has likely experienced overgrazing, or a wide area of heavily compacted soil without litter cover can imply heavy social use. However, the reason for a condition cannot be definitively determined, the condition is simply documented. 75 5.7.3 PARK-LEVEL CLIMATE VULNERABILITY ASSESSMENT METHOD The geospatial vulnerability assessment applies a climate-smart conservation action planning approach to identify strategic opportunity areas where park management can achieve significant improvements or protection from anticipated stressors. These opportunity areas exist along a spectrum depending on the type of plant community, exposure to various threats, and current conditions. Using the geospatial analysis, parks managers can locate highly vulnerable locations where intensive restoration efforts may be needed and less vulnerable areas where slight modifications to routine maintenance can achieve desired benefits. Environmental Vulnerability Index (EVI) Method The climate vulnerability assessment was summarized with three indices, an Environmental Vulnerability Index (EVI), a Social Vulnerability Index (SVI), and a composite climate vulnerability index named the Park Climate Vulnerability Index. Geospatial layers for two major climate risks (fire + drought) and vegetation community condition (as described in Section 5.7.2) were combined with even weighting to create the EVI. Fire was incorporated via crown fire probability and drought incorporated via soil water availability. The two climate risk layers indicate degree of exposure, while the vegetation community condition rating incorporates sensitivity and adaptive capacity, which combine to create a vulnerability index of abiotic and biotic environmental factors. A poor vegetation community condition score was a higher sensitivity and lower adaptive capacity condition resulting in higher overall climate vulnerability. Flooding was not included in the EVI as it is often a counter-indicator to the other climate risks and suggests a more discrete zone and set of management activities. Extreme heat was also not included in the EVI as it too was very localized in scale and considered more of an exacerbator of wildfire and drought than a fundamental climate risk for vegetation communities. Social Vulnerability Index Method Additionally, social equity considerations were incorporated using the CDC’s SVI. As noted in Section 3.2, the SVI uses 16 census tract variables to assess the capacity of communities to prepare for and recover from significant disruption such as wildfire and other climate hazards. Areas of high SVI also often correlate to spatial patterns of inequity in historic and/or current levels of investment, economic opportunity, and access to resources. Park Climate Vulnerability Index Method The EVI and SVI were combined with even weighting to create the Park Climate Vulnerability Index. This composite climate vulnerability index evaluates environmental and social vulnerability and resilience, identifying potential needs to assist with prioritization and planning. 76 5.7.4 GEOSPATIAL VULNERABILITY ASSESSMENT RESULTS Environmental Vulnerability Index Results Figures 5-15 and 5-16 present the EVI results for the system and for Trevino. Figure 5-15. Environmental Vulnerability Index for PARD lands within study area. The index shows the combined risk layers for crown fire, heat and soil water availability . 77 Figure 5-16. Environmental Vulnerability Index for Trevino Metro Park as an example of site scale mapping. As shown in Figure 5-7, crownfire probability is highest in the northeast in the Decker complex of Louis René Barrera Indiangrass Wildlife Sanctuary, Decker Tallgrass Prairie Preserve, Water E. Long Metro Park. Additional high crownfire probability areas are scattered through several parks throughout the system. Soil water availability (Figure 5-10) has a distinguishable east-west pattern with deeper Blackland Prairie soils in the east that have higher soil water availability and generally lower drought hazard, and shallower Edwards Plateau soils in the west with lower soil water availability and generally higher drought hazard. The vegetation community condition scoring (Figure 5-13) has some clustering of poor condition/ high vulnerability conditions in the east along the Colorado River but is otherwise rather 78 heterogeneous throughout the park system. These patterns of crownfire probability, soil water availability, and vegetation community condition combine in the EVI. In general, the east has lower EVI levels, and the west has higher EVI levels, but there is also significant variation of EVI within each park. The most extensive areas of highest EVI are present in Louis René Barrera Indiangrass Wildlife Sanctuary in the east and Barton Creek Wilderness Park and Emma Long Metro Park in the west. Social Vulnerability Index Results Figures 5-17 and 5-18 present the SVI results for the system and for Trevino. The SVI in Austin exhibits a distinct east-west pattern, with higher social vulnerability generally concentrated in the east, and lower social vulnerability in the west. Southern Walnut Creek Greenbelt, East Boggy Creek Greenbelt, and Colorado River Park Wildlife Sanctuary are located in census tracts with the highest social vulnerability ratings. Other parks in high social vulnerability areas include Louis René Barrera Indiangrass Wildlife Sanctuary, Decker Tallgrass Prairie Preserve, Water E. Long Metro Park, Big Walnut Creek Nature Preserve, Red Bluff Nature Preserve, Old San Antonio District Park, Wunneburger Neighborhood Park, and Old San Antonio Greenbelt. 79 Figure 5-17. Social Vulnerability Index for PARD lands within study area. The index shows the combined variables that identify the differing levels of potential vulnerability at the census tract level. 80 Figure 5-18. Social Vulnerability Index for Trevino Metro Park as an example of site-scale summary mapping. 81 Park Climate Vulnerability Index Results The combination of EVI and SVI finds the highest combinations of environmental and social vulnerability, which create a heterogenous pattern throughout the park system. Figures 5-19 and 5-20 are the outputs of the Park Climate Vulnerability Index. The most extensive areas of highest Park Climate Vulnerability are located in Louis René Barrera Indiangrass Wildlife Sanctuary and Southern Walnut Creek Greenbelt in the northeast and Barton Creek Wilderness Park and Mary Moore Searight Metro Park in the south. Figure 5-19. Park Climate Vulnerability Index combining environmental and social vulnerability in the study area. 82 Areas of highest Park Climate Vulnerability are also present in Decker Tallgrass Prairie Preserve, Walter E. Long Metro Park, Colorado River Park Wildlife Sanctuary, East Boggy Creek Greenbelt, Red Bluff Nature Preserve, and Colorado/Walnut Greenbelt in the east; Barton Creek Greenbelt and Gaines Greenbelt in the west; and Old San Antonio District Park in the south. Figure 5-20. Park Climate Vulnerability Index combining environmental and social vulnerability as an example of site scale mapping. 83 The bar graph in Figure 5-21 Summary Ranking of Park Climate Vulnerability Index summarizes the index on an area-weighted basis for each park boundary to give a summary park level score. Under this summary approach, the full range of the index values within each park and the amount of area that they cover affect the park level score. Parks that scored above 3.5 in descending order are Colorado River Park Wildlife Sanctuary, Louis René Barrera Indiangrass Wildlife Sanctuary, East Boggy Creek Greenbelt, Barrow Nature Preserve, Decker Tallgrass Prairie Preserve, and Old San Antonio District Park. Both the identification of parks with highest Park Climate Vulnerability present, the total area of that highest vulnerability, and the summary park level scoring should be considered in management decisions and prioritization. 84 Figure 5-21. Summary Ranking of Park Vulnerability Index for PARD lands in study area. 6 Land Management 6.1 OVERALL MANAGEMENT APPROACH The parklands in this plan contain a variety of community types and conditions. The structural and compositional management target for a given parcel depends on a wide range of variables. Management decisions begin with an assessment of target community or site ecological condition and generally seek to improve overall condition and function. The following target communities are appropriate across the majority of the lands in this plan, serve multiple goals, and can be thought of as broad categories to be modified as necessary by site-specific considerations. The dominant community types, management histories, current conditions, adjacent land uses, and other variables constrain what is feasible and sustainable as a desired vegetative condition for each unit (see Prioritizing Implementation). The strategies most appropriate for each site are influenced by a suite of factors such as fire risk or community context, which can be layered to find opportunities for multiple benefits from management interventions. The menu of possible strategies is informed by local conditions and a few overarching principles of ecological planning. 6.1.1 TARGET COMMUNITIES General best management approaches for each target community are described below, and Table 6 contains suggested condition classes for common community types found within Austin PARD lands. Mixed woodlands Manage understory to achieve an open timber structure to improve drought and heat resilience, improve diversity, and reduce the probability of crown fire. Maintain diverse canopy, midstory, and herbaceous layers and low invasive species cover. Juniper-oak woodland Increase hardwood cover to at least 30% through species addition, strategic canopy opening to allow hardwood release, and protection from deer herbivory. Maintain a diverse age structure. In Golden- cheeked warbler habitat, or potential habitat, align management with the Balcones Canyonlands Preserve (BCP) Land Management Plan, follow BCP regulatory requirements, and restrict significant vegetation maintenance to non-nesting season (September 1-March 1). Grassland Grassland may encompass a variety of vegetation types, such as prairie, savanna, or open meadows, existing along a canopy cover gradient from near zero up to about 30%. Species richness and diversity of herbaceous vegetation are high. Canopy cover in grasslands is typically determined by a variety of site level as well as social factors. However, in the absence of strong abiotic regulators such as soil type, high input of management resources is often necessary to maintain grasslands, especially with canopy cover near or much above 30% without the system transitioning to a shrubland or woodland state. Protect existing reference species and increase diversity with species addition as appropriate to the site. 85 Bottomland Gallery mixed hardwood forest or mesic grassland type community, often existing along a moisture gradient from riparian to upland. Contains a diverse assemblage of obligate and facultative wetland species as well as a high percentage of woody and herbaceous stabilizer species. Perennial or near- perennial streams typically are protected by riparian buffers at least to the top of the first flood terrace. Ephemeral streams or small (first or second order) streams high in a watershed may not, however, enable the moisture regime to support wetland or even mesic species. Therefore, it is appropriate for many such streams to be vegetated primarily by dense herbaceous vegetation such as tallgrass or grassy open woodlands. Table 7 Community Condition Classes. This table can also be found in Appendix 3. 86 Very goodGoodFairPoorVery PoorHardwood cover>30%>30%15-30%1-15%0%Species DiversityHighModerateModerateLowVery lowAge class distributionGoodGoodModeratePoorPoorBare ground<5%<5%<5%5-30%>30%Erosion severity/extentLow/LowLow/LowLow/LowMod/ModHigh/HighFuel modelTimberTimberShrubShrubShrubCanopy species4+<4<3<3<3Species DiversityHighHighModerateLowLowAge class distributionGoodGoodModeratePoorPoorHerbaceous cover>50%25-50%5-25%<5%<5%Invasive sp cover<5%<25%<25%25-50%>75%Bare ground<5%<5%<5%<5%>5%Erosion severity/extentLow/LowLow/LowLow/LowLow/LowMod/ModFuel modelTimberTimberShrubShrubShrubCanopy cover<10%10-30%30-50%>50%>50%Species DiversityHigh. Conservative sp presentHigh/Mod. Conservative sp present, native increasers dominate.Moderate. Conservative sp absent. Native grasses primarily increasers.Low. Conservative species absent.Low. Conservative species absent.Forb cover/diversity>50%/High26-50%/Moderate<20%/Moderate<20%/Low<20%/LowInvasive sp cover<10%10-25%25-50%50-75%>75%MicrotopographyPresentAbsentAbsentAbsentAbsentBare groundAppropriate to ecositeAppropriate to ecositeAppropriate to ecositeHigher than ecositeHigher than ecositeErosion severity/extentAbsent/AbsentAbsent/AbsentLow/LowMod/ModHigh/HighFuel modelGrassGrassGrassShrubShrubCanopy cover>50%>50%>50%>50%>50%Herbaceous cover>75%50-75%VariableVariableVariableSpecies DiversityHighHighModerateLowLowInvasive sp cover<25%25-50%50-75%>75%>75%Riparian bufferto 25-year floodplainto 25-year floodplainto 25-year floodplainto 25-year floodplain<25-year floodplainErosion severity/extentLow/<25%Low/<25%Low/25-50%Moderate/50-75%High/>75%Channel-floodplain connectivityConnectedConnectedConnectedConnectedDisconnectedCanopy cover<30%<30%<30%<30%<30%Herbaceous cover>75%>75%50-75%VariableVariableSpecies DiversityHighHighModerateLowLowInvasive sp cover<25%25-50%50-75%>75%>75%Riparian bufferto 25-year floodplainto 25-year floodplainto 25-year floodplainto 25-year floodplain<25-year floodplainErosion severity/extentLow/<25%Low/<25%Low/25-50%Moderate/50-75%High/>75%Channel-floodplain connectivityConnectedConnectedConnectedConnectedDisconnectedBottomland grassland/ riparianBottomland woodland/ riparianPlant communityConditionParameterOak-Juniper woodland. (Canopy cover >30%. Juniper cover > 50% of wooded component)Mixed woodland. (Canopy cover > 30%. Juniper cover <50% of wooded component)Grassland - Savanna, prairie. Woody cover <30%.6.1.2 FUEL TYPES Fuel types will be referenced throughout the report. These are categories of fuel models (Anderson 1982, Scott and Burgan 2005) used broadly by wildland fire professionals to understand and model fire behavior in vegetation types throughout the U.S. The five general categories of fuel models referenced in this plan were drawn from the CWPP and are listed below. ● Timber: Wooded community with separation between surface and canopy fuels and typically an open understory. Fire does not readily move from the surface to the canopy. Canopy fire is typically isolated torching. ● Shrub: Wooded community with connected surface and canopy fuels. Fire may readily move from surface to canopy fuels and may be extensive under certain conditions. The understory is often dense. ● Grass: Continuous surface fuels consisting primarily of grass and other herbaceous plants. Canopy cover is typically low but may be high in the case of mixed woodlands with open canopies and continuous grassy understories. ● Slash (cut woody material): A layer of patchy or continuous cut woody vegetation, usually resulting from mechanical treatment such as selective thinning, brush control, or (elsewhere) logging operations. ● Special: For the purposes of this plan, we reference a fifth fuel type to identify isolated areas of standing dead fuels or dense live fuels. Often caused by drought. 6.1.3 FIRE RESILEINT LANDSCAPES A healthy ecosystem is inherently a “fire resilient landscape.” The plant community condition assessments and Environmental Vulnerability Index indicate that many of the natural areas considered in this document are currently, or will be in the coming years, highly vulnerable to drought and extreme heat, which together exacerbate community-level wildfire risk. Therefore, implementing landscape scale restoration treatments will be necessary to address the biotic landscape components that contribute to wildfire risk to both structures and natural resources. Fuel reduction treatments of all types such as shaded fuel breaks, selective thinning treatments, dripline treatments, understory mastication, or any other type of management action that restores healthy woodland structure can be important for reducing the likelihood of canopy fire and mitigating fire risk. While additional resources will be needed to implement landscape-scale woodland health treatments, PARD and AFD currently have mechanisms in place for implementing shaded fuel breaks or other types of boundary fuel treatments. These mechanisms include: - Written best management practices for shaded fuel breaks and other boundary fuel treatments in the Austin area, including in endangered species (Golden-cheeked Warbler) habitat - A scope of work for implementing shaded fuel breaks or other boundary fuel treatments - Contracts for implementation of shaded fuel breaks or other boundary fuel treatments - Funding source from Austin Fire Department 87 - Interdepartmental Agreement between PARD and AFD for wildland fire collaboration that addresses: o Planning of fuel mitigation projects and prescribed fire activities o Fire prevention and education activities o Fire response and recovery o Wildland staff training o Standards and procedures each department will use when mutually engaged in these activities Evaluation criteria for potential boundary fuel mitigation projects Prioritized list of potential boundary fuel mitigation projects - - - Methods for data recording and reporting - AFD staff to provide outreach, education, and risk assessments related to wildfire prevention and preparedness (e.g. FireWise principles) on private property All management recommendations discussed in the following sections are in alignment with the Austin/Travis County Community Wildfire Protection Plan: The National Cohesive Wildland Fire Management Strategy (the Cohesive Strategy) (USDA and DOI 2011a) identifies the following vision for national wildland fire management policy in the 21st century: “Safely and effectively extinguish fire, when needed; use fire where allowable; manage our natural resources; and as a nation, live with wildland fire.” The Cohesive Strategy recognizes three primary factors that will present the greatest challenges and opportunities for addressing national wildland fire problems and achieving the vision: 1. Restoring and maintaining resilient landscapes – Goal: “Landscapes across all jurisdictions are resilient to fire-related disturbances in accordance with management objectives.” 2. Creating fire-adapted communities – Goal: “Human populations and infrastructure can withstand a wildfire without loss of life and property.” 3. Responding to wildfires – Goal: “All jurisdictions participate in making and implementing safe, effective, efficient risk-based wildfire management decisions.” Programmatically within the City of Austin, there are processes and relationships in place to help achieve Factors 2 and 3 to address wildfire risk in the urban and interface areas. Unfortunately, there are not robust processes or resources in place to fully achieve Factor 1 in wildland areas, especially PARD natural areas. Indeed, this inability to achieve fire-resilient landscapes in Austin was the impetus for this plan. This plan is intended to guide the restoration and management of PARD natural areas so that when wildfires do occur, the fire behavior will be more manageable, the neighboring structures more defensible, and the natural areas able to recover largely on their own from a process that is, on balance, helpful rather than harmful. This will have a positive effect on adjacent private lands and will also help to ensure that parklands are able to continue serving the Austin community as climate change intensifies. 88 Shaded fuel breaks will be evaluated and prioritized as potential management treatments during the development of annual work plans for individual PARD management units. This will help to provide synergistic effects of different management actions to ensure cost effectiveness, optimal cost-benefit relationships, the achievement of multiple public benefits, and will ensure the City’s ability to maintain investments in management through periodic maintenance or continued restoration actions. Because of rapid regrowth of vegetation in shaded fuel breaks, they must be maintained on average every four to five years. Without this maintenance, the probability of canopy fire post-treatment can become higher than it was pre-treatment. Thus, integrated planning of fuel mitigation and other restoration efforts will be needed to prevent the loss of investment via deferred maintenance as well as to maintain risk reduction benefits of fuel breaks. 6.2 ECOSYSTEM SERVICES AND ECOSYSTEM FUNCTION Management recommendations are guided by general ecological principles of ecological planning which strive to support ecosystem services and functions. 6.2.1 ECOSYSTEM SERVICES As described in Sections 1 through 3, healthy natural communities provide ecological services such as human physical health, mental health, and social well-being and environmental products and services such as clean water, clean air, temperature moderation (reduced urban heat island), wildlife habitat, and research and education opportunities. The ability of a natural system to provide ecosystem services depends on its level of ecological function, or ecological health. Highly functional, or healthy, systems as described in the following subsections can provide a more robust suite of ecosystem services. 6.2.2 ECOSYSTEM FUNCTION Three primary processes occur in all ecosystems: energy capture through photosynthesis, nutrient cycling, and a set of hydrologic processes. The efficiency of these processes, and the degree to which they are under biological control, define the level of function, or health of the ecosystem (Whisenant 2002). Ecosystem function can be thought of on a spectrum (Figure 6-1). Highly functional communities can efficiently capture and retain resources, and self-repair following disturbance. The biotic portion of the system exerts control over resource flows – enhancing water capture and infiltration, driving nutrient cycling, and retaining soil. Communities with low levels of function are often unable to recover following disturbance and lose resources such as soil, water, and nutrients. The goal of land management is to remove barriers to ecosystem function. Community type and condition influence the details of how these processes operate. 89 Figure 6-1. Ecosystem function. Stepwise degradation of hypothetical natural community illustrating two common transition thresholds that separate the three vegetative groups emphasized here. Their functional integrity and transition limitations rather than species composition define these groups. Natural communities controlled by biotic interactions require some form of vegetation manipulation before recovery can occur. Transition thresholds controlled by abiotic limitations require physical manipulations that increase infiltration, reduce erosion, capture organic materials and/or ameliorate microenvironmental extremes. Adapted from Whisenant 2002. Species diversity & structure Species diversity is a critical component of ecological function and health. Diverse communities are better able to withstand disturbances such as disease and insect invasion, because only a portion of the community is affected at a time. Diverse communities can adjust to changing conditions because the likelihood is higher that a species adapted to the new condition is present. Additionally, individual species play functional roles within the community such as soil building, bringing nutrients from deep in the soil column to the surface, and fixing nitrogen. Diverse communities are able to perform more functional roles, are more likely to have species present that are efficient in their role and have some redundancy of roles which lessens the threat to the community if one species is lost. Diversity of strata provides habitat for a variety of animals, with some such as forest arthropods spending their life restricted to one strata, while others such as birds and squirrels range through the strata. The ground layer, and just below it, supports the greatest diversity of life (Smith 1996). Many species such as soil and litter invertebrates remain in the upper layer of soil while others burrow deeper. Nutrient cycling Nutrient cycling may be considered as a balance between inputs to the biological system and outputs from the system. Nitrogen is an important nutrient to consider. Forest communities tend to accumulate and cycle large amounts of nitrogen. Most of the nitrogen is incorporated in the mineral soil horizons and the remaining is in vegetation and the forest floor. Deciduous and coniferous forests differ in the magnitude and nature of nutrient cycling. More nutrients are cycled through deciduous forests than 90 coniferous because conifers retain more nutrients in their biomass, rather than dropping some with leaves every year. The distribution of nitrogen and organic matter also differs between old and young stands. Older stands tend to have larger total biomass, higher litter, and soil organic matter (Smith 1996). The distribution of nitrogen in grasslands differs from forest systems, as does the speed of nutrient cycling in general. In grasslands, 90 percent of nitrogen is bound in soil organic matter, 2 percent in litter, 5 percent in live and dead plant cover, 1 percent in dead shoots, and 0.8 percent in soil microflora (Smith 1996) The accumulation of detritus is central to nutrient cycling in grasslands, and plant consumers are particularly important. Invertebrate consumers convert ingested material to feces, and they are rapidly returned to the system. The dung of large grazers is an important nutrient cycling pathway, concentrating nutrients from a large area. A large accumulation of thatch can have a detrimental effect on nitrogen cycling (Knapp and Seastedt 1986), as it inhibits nitrogen fixation by soil microbes and intercepts rainfall. Periodic fires clear the thatch and stimulate the growth of nitrogen fixing leguminous forbs. The carbon cycle is strongly influenced by natural communities. Energy flow is often measured in terms of carbon pools and fluxes. In wooded communities, trees absorb carbon dioxide through photosynthesis, release it through respiration, and retain some as part of biomass. Some of this biomass drops to the ground as litter which decays and is released back to the atmosphere or becomes part of soil carbon. Similarly, when trees die, some carbon is released into the atmosphere and some returns to the soil. Forest biomass includes aboveground biomass, belowground biomass, dead wood, litter, and soil organic carbon. Live and dead trees store 50-60% of the carbon in forest communities (McKinley et al. 2011) and soils store roughly 16% (Sedjo and Sohngen 2012). Although forests release carbon into the atmosphere through respiration and plant death, they are considered overall carbon sinks. Carbon budgets differ between woodland and forest types. Carbon accumulation in grasslands occurs primarily below ground (Soussana et al. 2004). Carbon is stored in the soil as organic matter or humus. Carbon is sequestered in the soil through humification as plants die and decompose creating organic soil matter. The organic matter is further decomposed into the stable form humus. Microphotosynthesis is a second process through which carbon can be stored in soils. Photosynthetic bacteria within the soil sequester CO2 from the atmosphere. Soil can store carbon for a long time, while aboveground storage is more vulnerable to disturbances that kill trees (Birdsey et al. 2019). Communities differ in their above and below ground storage capacity (Figure 6-2). 91 Figure 6-2. Organic carbon density above ground (green bars) and below ground (brown bars) is dependent on ecosystem. Adapted from (Amthor et al. 1998). Disturbance and Natural Processes A disturbance is a discrete event that disrupts the structure, community, or population within an ecosystem (Turner et al. 2001). Disturbance regimes influence species composition and structure and thus are important for regulating competitive dynamics and establishing heterogeneity in ways that maintain diversity and ecosystem function. Natural disturbances such as insect kill, fire, flooding, winter/ice storms, drought, herbivory, and animal impact create a patchy landscape with spatial diversity, species diversity, and age diversity. Disturbances may be discrete events, such as a single fire, wind event, or ice storm, or periodic or on-going processes, such as continuous grazing or frequent, regular flooding. Some important components of disturbance regimes are defined below (Turner et al. 2001): Frequency: Mean or median number of events occurring at a location per time period, or decimal fraction of events per year; often used for probability of disturbance when expressed as the decimal fraction of events per year. Example: 0.2 fires per year, 20 fires per 100 years. Intensity: Physical energy of the event per area per time (e.g. unit of heat released per area per time period for fire, or wind speed for storms) Return interval: Mean or median time between disturbances; the inverse of frequency; variance may also be important, because this influences predictability. Example: 1 fire every 5 years, 1 flood every 100 years. Severity: Effect of the disturbance event on the organism, community, or ecosystem. Closely related to intensity, because more intense disturbances generally are more severe. Example: 20% top-kill or 6 ft scorch height of a fire, 10% removal of a woodland thinning treatment. 92 Size: Area disturbed, which can be expressed as mean area per event, area per time period, or percent of some study area per time period. The most significant disturbances that historically influenced Austin-area ecosystems include fire, herbivory (grazing by bison, browsing by deer), flooding, and pest/disease kill. Periodic drought is a region-wide disturbance that influences community composition and structure and can change the frequency and severity of other disturbances such as fire. Following this general discussion, these disturbances will be discussed in the context of each community type. Changes in fire regime shift a community’s composition and structure. Fire frequency, season, and intensity all influence how the community responds post-fire. For example, woodlands with frequent, low intensity surface fire tend to have an open structure, well-developed herbaceous layer, a high proportion of fire tolerant species, and lower loading of dead and down wood. Low-intensity fire often creates high spatial heterogeneity within communities because it tends to act in a patchy fashion, with some areas more severely affected than others. Conversely, wooded systems without a history of fire or with very infrequent, patchy fires tend to be dominated by species that are fire intolerant, have a denser structure (high density of individual trees as well as high biomass of live canopy and dead wood), and a reduced herbaceous layer. Wooded systems with this kind of structure and composition are often resistant to all but the most intense fires and often experience long fire-free periods until a canopy-level stand replacing fire occurs. Recovery to near pre- fire conditions following this type of fire occurs over very long time periods. Thus, in such forests without recent fire history, reintroduction of management burns often first requires mechanical manipulation of stand structure to allow for more frequent, low-intensity burns. In grasslands, fire retards woody encroachment, plays a role in nutrient cycling, stimulates new growth in grasses and temporarily removes thatch. In its absence, grasslands with enough water to support trees will begin to convert to woodland communities. Herbivory and fire are often linked. Young or resprouting vegetation tends to be more nutritive and palatable. Therefore, grazers and browsers often prefer to feed in newly burned areas. Herbivory may also alter fuel characteristics in ways that change fire behavior. With respect to Austin natural areas, intense browsing and altered fire regimes most significantly affect recruitment of conservative hardwood species such as Texas red oak (Russell 2001). Grasslands evolved under grazing pressure from ungulates since the Cenozoic and have adapted to this pressure. One response is compensatory growth in which remaining tissue increases photosynthetic rates and nutrients and photosynthates from roots to stems. Removal of photosynthetically inefficient tissue makes room for young tissue added to the increase in light stimulates growth. Moderate grazing stimulates primary productivity in grasslands (Dyer et al 1993). Heavy grazing leads to a loss in primary productivity and eventually species compositional change. Insect and disease kill is a natural and important part of forest and grassland function. Individual dead trees or shrubs can serve as important components of wildlife habitat and their open canopy gaps become locations for competitive release of new individuals or species. Loss of individuals to insects or disease helps create uneven age structure and facilitate adaptation by removing the most vulnerable individuals. This type of baseline mortality generally is important for the development of diverse 93 communities with multiple age classes. Pests and diseases become problematic when they cause widespread mortality that is significantly higher than baseline rates. Further, as communities are stressed by changing conditions, they may be less able to recover from otherwise normal or natural disturbances. 6.3 MANAGEMENT ACTIONS BY COMMUNITY TYPE 6.3.1 WOODLAND MANAGEMENT Woodlands and Forests Woodlands and forests are often discussed as distinct types of wooded vegetative communities. Although there are no standard and agreed-upon definitions of each, and indeed the terms may often be used interchangeably, they are defined here for the purposes of this document. Woodlands are usually characterized by open-growth trees, a general absence of a true midstory canopy layer but often containing a well-developed understory and surface layer of herbaceous and woody plants. Wooded systems existing within a larger grassland ecoregion (for example, riparian woodlands in the Great Plains) may also be termed woodlands. In Central Texas, the term “woodland” is often used preferentially in reference to the relatively short stature of trees and shrubs in the area, rather than in reference to density or light conditions. Conversely, forests usually have closed or nearly closed canopy, and typically have a true midstory canopy layer. Most forest understory species are adapted to low light conditions, and the herbaceous layer is often sparce or almost non-existent except for short times of the year, such as early spring when a diversity of herbaceous species may flower and set seed. The term “forests” can also be used to describe wooded systems that are geographically expansive enough to be considered ecoregions, such as the East Texas Pineywoods. In this section and throughout this document, the terms woodland and forest are used in reference to the accepted names of vegetation types (e.g. “oak-juniper woodland,” “gallery forest”). Elsewhere in this document, we will either use the terms interchangeably or use other terms that are more specific in their meanings (e.g. “shrub” or “timber” fuel models). Many forests and woodlands in the Austin area are relatively young second- or third-growth systems that differ from old growth (plant communities with little history of significant human disturbance), though old-growth communities do exist. Old-growth stands are dominated by very large trees, tend to have a more open and diverse structure than younger communities, and are characterized by complex relationships between organisms (Smith 1996). Old growth juniper woodlands often approach the structure of a true forest and are best matched by a “timber” fuel model. Highly developed, uneven-aged forests and woodlands usually consist of three or four strata. The upper canopy and sometimes lower canopy (overstory), understory sapling trees and shrubs (the midstory) and a ground layer that can consist of a mix of low herbaceous and woody species. Basic woodland/forest ecology Structure 94 Stratification influences microclimates with the highest temperatures found in the upper canopy and decreasing through the lower strata. Humidity tends to be high in the interior in the summer because of plant transpiration and poor circulation. This, along with lower temperatures, plays a role in reducing the likelihood of fire within developed forests and woodlands. Diverse age structure is also important to community function and resilience (O'Hara and Ramage 2013). Even-age stands and simplified structure often result following clear-cutting, changes in land use, and changes in natural disturbances. This can also occur in converted communities such as grasslands undergoing woody encroachment or newly colonized cultivated or highly disturbed land (go-back land). These communities provide fewer habitat opportunities and are less resilient overall. Diverse age structure is important to ensure replacement of aging individuals. Also, single age stands become more susceptible to disease and pests at the same time. Pests/disease of concern Although arboricultural interventions are available and commonly used in parks to treat important or valuable specimen trees, at large scales, performing targeted disease treatments on individual trees or groups of trees is usually not feasible, economical, or even effective. In natural areas, pest and disease management typically involves managing for and maintaining structurally and compositionally diverse vegetative communities. This reduces the density and increases the separation distance of individual trees of susceptible species. Thus, although a few individuals may succumb, the spread of the disease is reduced or slowed, or at worst, other resistant species are able to maintain community structure and function if susceptible species are dramatically reduced. Pests and diseases of concern in the City of Austin include (Brandt et al. 2020): • Oak wilt (Bretziella fagacearum) • Hypoxylon (Biscogniauxia atropunctatum) • Emerald ash borer (Agrilus planipennis) • Dutch elm disease (Ophiostoma novo-ulmi) • Bacterial leaf scorch. (Xylella fastidiosa) Principles of woodland/forest management Most uplands within the scope of this document currently support wooded communities. Generally, maintaining or enhancing species diversity, age diversity, and internal spatial heterogeneity, as well as encouraging an open structure and surface-to-canopy fuel separation will help communities become more resilient to pests, disease, wildfire, and changing conditions (Swanston et al. 2016, Anderson et al. 2018, Stambaugh et al. 2021, Sample et al. 2022). These concepts will be discussed in the context of the Ecoregions and community types contained within the project below. The woodlands and forests of the Edwards Plateau and Blackland Prairie are part of a larger trend in both eastern and western forests of densification and compositional shift (Pellegrini et al 2021). Oaks are failing to regenerate in many regions and in Eastern forests the trend is toward more mesic species (Abrams 1992, Nowacki and Abrams 2008). In the drier Edwards Plateau, the shift is toward increased juniper dominance (Andruk et al 2014, Diamond and True 2008, Murray et al 2013). Fire exclusion over the last century has contributed to densification and the reduction or loss of fire adapted communities 95 (Stambaugh et al. 2021). Intense herbivory by white-tailed deer (Odocoileus virginianus) deer is contributing as well (Andruk et al. 2014). The loss of hardwood diversity reduces the habitat value of the woodland and resilience parameters related to diversity, but it also changes how the community is able to respond to fire. Most woodlands within the Blackland Prairie ecoregion are mixed woodland or bottomland community types. Some are oak-juniper dominated, but deciduous elm-hackberry woodlands are also common in the uplands as well as mesquite-midgrass communities. Management goals are similar to those within the Edwards Plateau - uneven aged stands, high diversity in all layers, Timber fuel model - but some conditions differ. The Blackland Prairie ecoregion typically has deeper soils and higher water availability, simplifying species addition. Unfortunately, these conditions also facilitate colonization by invasive species. Within the Blackland Prairie ecoregion, invasive species were less dominant in closed canopy woodland than in open areas. Woodlands in the Blackland Prairie ecoregion also tend to have a very limited native seed bank, necessitating active addition of species. Species addition and midstory thinning is recommended, but opening the overstory canopy should be used with caution because of high invasive pressure. Oak-juniper woodland is not receptive to fire under most conditions (White 2009). During an 8-year period that contained the current drought of record in 2011, McCaw et al. (2018) found that Ashe juniper in the Austin area had live fuel moisture sufficiently low enough to allow for extensive crown fire approximately 6% of the time. However, as climate change progresses, fire frequency and/or intensity in this community type is likely to increase. With the exception of juniper species, most woody species native to the Edwards Plateau are able to resprout following top-kill by fire. Following crown fire in a juniper dominated woodland, a variety of species such as live oak, shin oak, red oak, sumac species, and many others vigorously resprout to create a “shinnery” or shrubland of dense, short, trees and shrubs. As juniper does not resprout, it is often absent from the shrubland for many years until it recruits back to the area from seed. This dynamic has been documented more recently as well (Reemts et al. 2019). This recovery following a crown fire is predicated on a complement of fire-adapted species being present, with sufficient cover, in the woodland prior to the fire. Adams (2013) argued we are on the cusp of fire-driven ‘tipping points’ in woody biomes that could promote significant changes in species dominance. “Megafires” are positive feedbacks to changing climates via carbon emissions. A contributing factor is human management of wooded ecosystems that has allowed high fuel loading. Local evidence of this was seen in the 2011 Bastrop fires in which high fuel loads, even-aged stands resulting from former clear cutting, combined with fire conducive conditions to create very high intensity canopy fires that have been followed by slow recovery. Many of Austin’s woodlands have high fuel loads, even-aged stands, and dense midstory. . Many woodlands have lost, or not yet developed, species and structural diversity. Mature hardwoods are often present, but are not recruiting new individuals, as evidenced by the lack of seedlings and saplings (Russell 2001). Juniper is regenerating, but its high stem density and canopy biomass has increased competition for resources and reduces community-level species and spatial diversity. Increased woodland resilience and reduced risk of crown fire can be encouraged through restoration of natural processes, such as fire, by reducing density and increasing surface-canopy separation 96 (Greenberg & Collins.2021, U.S. Department of Agriculture-Forest Service 2022). Researchers working in the western United States responding to existing and expected increases in the area burned and severity of wildfire within seasonally dry forests have argued that prescribed fires and restoration thinning strategies provide benefits including enhanced biodiversity, increased water availability, protected carbon pools, and more sustainable carbon sequestration (Stephens et al. 2020). These strategies, coupled with species addition, reduce the risk of crown fire while improving overall community resilience (Greenberg and Collins 2021). The CWPP identifies several treatments for fuel mitigation that can also improve overall resilience, these include: o Dripline thinning o Selective thinning ▪ The “dripline” is the area at the end of the longest branches of a tree or shrub where water drips vertically to the forest floor. Dripline thinning is accomplished by removing ladder fuels and competing small brush and trees from within the dripline circumference of desired “leave trees.” The technique of dripline thinning can be used to reduce crown fire potential and relieve desired trees from competition for nutrients, sunlight, and water. ▪ Trees or brush within a forest or woodland may be mechanically thinned according to a prescription for target species, size class, density, or other factors to help shift structure, fuel loads, or species composition. Thinning is a means of improving forest health and promoting long-term viability of some fuel types and forest regimes. Thinning encourages trees and community composition that are more heat, drought, and disease resistant. Thinning is often required as a first step to reducing high fuel loads, tree density, and increasing species diversity. It may also benefit more mature stands by reducing competitive stress of large trees. o Mastication ▪ Also referred to as mulching or “forestry mowing.” Mastication is utilizes light machinery to quicky remove dense, small-diameter trees and brush. Mastication is still considered a selective management technique, but is less selective than hand-cutting. Best used in areas with very high density of small- diameter target trees on relatively flat terrain. o Prescribed burning ▪ Broadcast prescribed burns use fire as a tool across a management unit to reintroduce the positive effects of low to moderate intensity fire. Improves diversity, reduces fuel loads, and improves fire resilience. May be used to consume slash created during cut-and-scatter selective thinning treatments. ▪ Pile burning is a technique for consuming slash created during cut and pile selective thinning treatments. ▪ All prescribed burn operations in the City of Austin are conducted with burn permits from the Austin Fire Department and follow the standards of the National Wildfire Coordinating Group and/or Texas Prescribed Burning Board. o Chemical treatment of invasive species or problematic resprouting native species ▪ Invasive plant species too large to be effectively removed by hand or mechanically must typically be treated with herbicide 97 ▪ All herbicide application in the City of Austin is conducted by Texas Department of Agriculture-certified pesticide applicators following departmental Integrated Pest Management plans. o Shaded fuel break ▪ Shaded fuel breaks are a type of fuel treatment in which woodlands or forests are limbed and/or thinned to minimize fire movement from the surface to the crowns. They have some effect on reducing ember production but are primarily intended to minimize the development of crown fires immediately adjacent to structures and increase fire suppression effectiveness. They can be used adjacent to homes but could also be located in other areas to strategically limit fire spread. Depending on the direction of fire travel, they may limit the incidence of canopy fire but may not be completely effective in stopping canopy fires once they develop. Effective shaded fuel breaks create Timber fuel types in strategic locations, which are least prone to the occurrence of crown fires. The CWPP identifies objectives and benefits of fuel treatments that also meet land management goals, including: o Promote smaller, lower intensity wildfires o Reduce the potential for canopy fire o Decrease the potential for catastrophic wildfires that may put lives and property at risk and permanently damage vegetation regimes and ecosystems. o Reintroduce low-intensity fire o Contribute to the ecological processes and functions of local forest and plant communities Improve the health of vegetation most suited to the site o o Maintain and enhance native species diversity o Maintain and enhance wildlife habitat o Control invasive species Managing for spatial heterogeneity Spatial heterogeneity improves resilience of natural systems to a variety of stressors (Swanston et al. 2016, Anderson et al. 2018, Sample et al. 2022, U.S. Department of Agriculture-Forest Service 2022). Spatial heterogeneity allows for varied response to stressors such as drought and increases overall species and habitat diversity. Managers can create diverse conditions through varied thinning prescriptions and prescribed fire timing that mimic natural disturbance (Swanston et al. 2016, Sample et al. 2022). Units should have patches at different stages of recovery following disturbance. Additionally, natural heterogeneity should be enhanced by identifying unique communities and refugia within the unit and building their presence into management planning. Examples include mature Juniper-oak woodland which already have a timber type structure, areas that naturally have more access to water and have additional protection from fire such as moist canyons with diverse and mature woodlands, seep and cliff communities. Additionally, floodplain areas are good candidates for species addition. Under certain conditions, species addition can be successful without canopy thinning (O'Donnell et al. 2020) and should be considered in areas conducive to it. Areas with high risk of crown fire, combined 98 with a shrub fuel type can be prioritized for thinning. Mechanical thinning even without prescribed fire has been shown to moderate fire behavior (Johnston et al. 2021). Time is an important factor. Changes in biodiversity and composition often take place over long time periods. The concept of pyrodiversity, mixed burn severity over an area, has increased overall avian (Tingley et al. 2016) and pollinator diversity (Ponisio et al. 2016) that were detectable at 10 years, but not at 1 year post burn as communities continue to differentiate. Within Golden-cheeked Warbler (GCW) habitat in the Edwards, positive impacts of prescribed fire on vegetation species and structural diversity of an oak-juniper woodland were seen after a six-year delay (Reidy et al. 2016). In this study, GCW showed an initial reduction of use of burned areas, but eventually returned unless fire severity was high. GCW ultimately showed the highest probably-to-use in areas with moderate severity (canopy intact but some intermediate level subcanopy mortality) and the lowest where fire severity was high. The reduction in warbler density appeared to be the result of avoidance of areas with high burn severity, where areas of low to moderate burn severity had high warbler use. Quantifying and defining the spatial heterogeneity for an area is challenging, but historic disturbance patterns and distribution provides some insight. Increased woodland health can be encouraged through restoration of natural processes, such as fire, or activities that mimic natural disturbances such as disease and insect kill which create canopy openings that vary in size, shape, and location. This encourages the woodland to move toward a state with mixed age stands, increased species diversity, and a diverse herbaceous layer. Thinning should be combined with species addition and, when possible, protection from deer. Tree architecture may also be modified to “limb up” trees simulating the effect of low intensity surface fires which kill lower branches of many woody species and promote herbaceous growth (CWPP). Invasive species management will be needed in all communities (see Appendix 4) and should follow the PARD Integrated Pest Management Plan and guiding documents such as the PARD Nature Preserves Integrated Pest Management Plan and the City of Austin Invasive Species Management Plan. Species which are more resilient to climate stress should be encouraged. Appendix 6 contains recommended species for addition. These species were selected based on a cross-walk of species documented on PARD properties with those identified as having low or moderate vulnerability in the Vulnerability Assessment of Austin’s Urban Forest and Natural Areas (Brandt et al 2020) Woodland Goals and Strategy Summary The goals and strategies for Woodlands are summarized in Table 7. Priority woodland strategies include invasive species management, selective thinning, fuel reduction, prescribed fire, species addition, and seedling protection. Invasive species documented during the site survey and a discussion of Integrated Pest Management can be found in Appendix 4. 99 Table 8 Woodland Goals and Strategies Selective thinning/fuels reduction Austin’s woodlands are part of a pattern in upland ecosystems of increased tree density and compositional shifts toward more fire sensitive species over time. Stambaugh et al. (2021) found that tree density is two to three times higher in contemporary forests than was recorded by land surveys in the 1800s. Reference communities within the appropriate ecoregion and condition set should be identified to ascertain appropriate thinning targets. Silvicultural retention thresholds between 10 and 30% showed higher overall species richness of birds, mammals, spiders and beetles, but lower amphibians than treatments with no thinning and clearcut treatments (Fedrowitz et al. 2014). However, interior forest species can be negatively affected. A conservative initial thinning target is reduction by 1/3 beginning with small diameter (e.g. <4” DBH) individuals of common or early-successional species as well as invasive species. 100 Thinning need not be evenly distributed throughout the treatment area. Creation of patches with varied thinning targets (up to 90%) and in varied stages of regrowth helps to create spatial heterogeneity and age diversity. Under natural disturbance, clusters of trees are often affected at the same time. This can be navigated by thinking in terms of multiple patches within the whole and an overall 75% retention rate. Some patches are thinned more aggressively and placed to influence overall fire behavior – at edges, to protect sensitive habitat during the event of a wildfire, and to create varied conditions throughout the woodland so that ensuring prescribed or wildfire will have varied intensity, thus furthering goals for spatial heterogeneity. Specialized management for specific conservation targets such as endangered species habitat management (GCW, BCV, cave invertebrates) or wetland management will need to be integrated into the larger strategy. At the park level – create or maintain patches. Treat patches in different years and to different intensities. Modify fuels to encourage this patchiness in follow up prescribed burns, or in wildfires when they arrive. The decision to remove or leave slash in place is based on volume and the option of fire as a management strategy. Moderate to low amounts of slash can be used to protect seedlings, distributed across the landscape, stacked along contours, piled for wildlife habitat, or chipped and distributed. Mulching treatments use machinery to masticate woody fuels and convert them to mulch. They have some effect on reducing intensities and movement of fire from surface to crown. They do not decrease the amount of heat at the surface. They increase the amount of tree mortality and slow recovery ratees and fire suppression in mulch fuels is problematic. Berryman et al (2015) found that distributed slash or masticated fuel at fuel loadings of up to 27 tons per acre in montane ponderosa pine forests in did not cause soil temperatures to exceed thresholds of soil biological damage under low intensity burns. Larger amounts of slash should be removed, or pile burned, and low slash loads can be broadcast burned. Note that if pile burning is used, piles should be kept small because the soil beneath will be damaged and natural revegetation will be slow. A revegetation strategy should be developed. Options for slash management (COA Watershed Protection woody debris management3, Austin/Travis County Community Wildfire Protection Plan4) include: • Scatter, following a prescription for slash placement and depth. This is the preferred method if broadcast burning is planned for the unit. Slash should be cut or broken into smaller pieces and scattered uniformly in a thin layer. 3 COA Watershed Protection Grow Zone Woody Debris Management. https://www.austintexas.gov/sites/default/files/files/Watershed/riparian/GrowZones-How-to- Manage-Woody-Debris-v2.pdf 4 Austin/Travis County Community Wildfire Protection Plan. https://www.austintexas.gov/page/austintravis-county-community-wildfire-protection-plan 101 • Pile, following a prescription for pile size and distribution. Windrow materials following contours in rows not exceeding 2 feet wide or tall, placed 10 to 20 feet apart. Do not place windrows on steep slopes adjacent to waterways, inside the high-water mark of a waterway, within 10’ of the edge of a waterway, or inside a drainage channel. Ensure a 30’ gap between windrows and active trails or infrastructure. • Chip when other options are not available or desirable. Do not chip fruits/seeds of invasive plant species as they may remain viable. o Chip and scatter with material no deeper than 1” o Chip and remove if the area is easily accessible and reduction of overall fuel load is desired Hand removal Hand brush removal involves the use of chainsaws or hand pulling by crews operating on foot. This approach can be a very effective method in areas that are sensitive or are difficult to access. Hand brush removal avoids the use of heavy equipment, does less damage to the soil structure, and can be more targeted than mechanical removal. However, it requires significant labor expense. Mechanical removal Mechanical methods are commonly used throughout Austin’s parklands today and involve the use of machinery such as skid steers to physically cut vegetation. Such methods should be implemented carefully to minimize soil disturbance. Such methods as mastication can be effective when used in conjunction with other brush control techniques outlined in this section (Hanselka et al. 1999). Preferred mechanical removal is the use of skid steer-mounted tree shears, which cut woody material at the surface without excessive soil disturbance, or mulching heads which grind plant material off above the soil surface. Avoiding the stacking of brush can substantially decrease soil compaction associated with repeated driving over the same ground to stack cut materials. Prescribed fire – moderate to low intensity Prescribed fire can be paired with mechanical thinning treatments to reduce fuel loads, increase species diversity, reduce mechanical treatment costs, and encourage fire-resilient landscapes. In shrub fuel types, mechanical treatment must often precede prescribed fire for the purpose of reducing canopy fuels and increasing fine surface fuels so that fire is able to carry across management units under prescribed moderate burning conditions. Fire return intervals will vary according to factors such as management goals, fuel conditions, and species composition. Typically, shorter return intervals are needed early in the restoration process. For example, in mixed woodlands and bottomlands, a fire return interval of approximately 6 to 12 years is suggested, although return intervals from 2 to 20 or more years may be appropriate for creating or maintaining an open structure with a well-developed herbaceous layer. In oak-juniper woodlands, similar return intervals (6 to 12 years) are suggested, where possible, for reducing juniper dominance and encouraging fire fire-resistant species, although much longer (30 years or longer) may be appropriate. Reidy (2021) found that low-intensity prescribed fire had slightly negative effects on Golden-cheeked warbler density and breeding success in the short term but provided beneficial effects to habitat structure in the long term (increased oak regeneration and decreased juniper seedlings). It should be noted that oak regeneration experienced a significant delay (6 102 years) post-fire. GCW preferred juniper dominated woodlands where a substantial amount of understory had been killed over areas experiencing less fire effects, though they avoided areas with canopy disruption. Moderate fire severity resulted in the most desirable changes in vegetation for reducing canopy fire risk and potentially increasing oaks and GCW made greater use of closed canopy woodlands with low or moderate fire severity. The biodiversity impacts of smaller, low intensity fire at intervals of 6 to 12 years to reduce fuels and mitigate fire size and intensity, need to be weighed against those of large-scale, high intensity wildfires at increasing frequency. Prescribed fire treatments should be paired with species addition and deer exclusion measures. Species addition and seedling protection Initially, select species to encourage or add that are native to the Edwards Plateau and the Blackland Prairie. Species documented during the site assessment that were rated as moderate or low vulnerability in the Vulnerability of Austin’s Urban Forests report (Brandt et al. 2020) are appropriate for encouragement. Additional species can be selected that have broad temperature and moisture tolerances, occur across a wide geographic area, or are found in a range of Habitats. See Appendix 6 for suggested species. Species addition strategies range from wildland restoration strategies in which a high number of seeds or seedlings are added to the system and receive minimal follow-up support. This method is inexpensive and allows treatment of larger areas. However, a survival rate of 30% is considered good. At the opposite end of the spectrum, horticultural methods can be employed in which fewer, often larger, specimens are installed and then are supported with supplemental irrigation. The appropriate method often depends primarily on funding and material. Tree Folks in Austin has had success with the former method, adding over 500 seedlings per acre, placed 8-9’ apart, during restoration efforts and providing no follow-up support. It should be noted that this was in denuded environments. Interplanting is more likely to be called for in this project. However, the addition of high numbers is still a good strategy if follow-up care is not planned. For a large forest enhancement project in north Texas, RES planted 2 to 3- foot-tall, containerized seedlings of a mix of hardwood tree species at 75 stems per acre. The goal of this planting was to increase age and species diversity, encourage desirable species, and replace invasive species that had been removed from existing mature forests. Hybrid approaches exist in which supplemental water is provided for a short time. Bare-root seedlings, container plants or salvaged material can be used to quickly revegetate the area. Tree plantings in particular can be very effectively undertaken with bare-root seedlings. If possible, when transplanting any of these types of materials, fill the hole with water, add the plant material, and then backfill, with the soil forming a muddy consistency. Field trials at the Lady Bird Johnson Wildflower Center have indicated that this method results in the greatest survivorship of the transplanted material, even if no subsequent water can be offered to the transplant. Cuttings may be useful in wetter areas. However, cuttings may not be the best choice in drier areas that are subject to extreme desiccation between flood events. Liners are an additional choice and often require less water for establishment. Seeding: The preferred seeding method is use of a no-till drill if terrain and tree density will allow it. Germination rates are higher than with hand seeding. 103 Seeding/installation windows: Seeding should be timed to take advantage of the spring (Late February- March) and fall (Late September-November) increases in rain. Seed can also be installed in the winter for delayed germination. Planting of seedlings should be done in the dormant period (December to February) to reduce shock and minimize water stress. Commercially available native seed can be utilized, and many species are available. Harvesting Seed Many species that may be unavailable commercially may be present on project lands and could be harvested. This is a good activity for volunteers, under supervision. Seed collection should follow ethical guidelines for collections so as not to diminish the natural spread of seed. Collection should occur only from well-established populations, collection should not occur every year from one population, and only a portion of available seed should be collected. Mechanical equipment is available for small-scale seed harvest. 6.3.2 GRASSLAND MANAGEMENT Grasslands are complex and diverse ecosystems that evolved under challenging conditions. Generally found in regions where rainfall is between 25 and 100 cm a year with frequent periods of drought. Climate, however, is not the sole driver of grassland formation. Frequent disturbance, in the form of fire and periodic grazing maintain grasslands, reducing woody species encroachment, playing a part in nutrient cycling, facilitating germination in some cases and encouraging new growth on the grasses, and increasing overall diversity (Smith 1996). Grassland are habitat to many species such as grassland birds dickcissels, quail, and painted buntings and many other plants, insects, and small mammals. Grasslands have declined significantly as they are converted to cropland or urban development or degraded by years of overgrazing. Grassland birds and other species that depend on the grasslands have declined along with the ecosystem. Grasslands are dominated by grasses and forbs. Amount of woody cover separates types of grassland on a spectrum, ranging from tree-free prairies to dense shrublands that are transitioning to forest. Prairie: Grasslands that are free, or nearly free, of woody cover. Savanna: Grassland with woody vegetation that ranges from a few scattered trees to about 30% woody cover. Shrubland: Shrublands are known by different names throughout the world and can be challenging to classify. Generally, shrublands have a scattering of shrubs with densely branched woody structure and low height, often with a near continuous herbaceous layer. Shrubland as a management goal is rare in the Austin area with the exception of Black-capped Vireo habitat on the Edwards Plateau. 104 Basic grassland ecology Structure Grasslands have three strata: roots, ground layer, and herbaceous layer. The root layer contains a larger proportion of biomass in grassland communities than other communities. The disturbance adapted species making up grasslands put more than half of their biomass underground where it is protected from fire and grazing. This biomass is arranged in roots that are concentrated in the first 16 cm of soil, but that extend down considerably. Little bluestem forms dense mats that extend to 1.7 meters (Weaver 1954) . Many species also produce rhizomes, underground stems, that can be used for reproduction and food storage. Long taproots that are able to reach deep moisture and nutrients are also common. The root zone itself has strata, often with three or four zones, so that species are able to absorb soil and nutrients from different depths within the soil that in roots and underground stems called rhizomes. The ground layer has low light intensity and is cooler than upper strata. It is inhabited by mosses and plants with rosette type growth form. Following grazing or fire, the conditions temporarily become warmer with higher light intensity. The herbaceous layer changes with the seasons. Small forbs on the ground layer grow early in the season. As the growing season progresses, taller plants fill in forming a middle and upper layer within the herbaceous layer. Grasslands accumulate a layer of thatch consisting of decaying residual herbage and standing dead herbaceous material from last season’s growth. It can take up to 3 or 4 years for this material to decompose. This layer conserves moisture, provides nesting cover for grassland birds, and offers cover for small mammals (Smith 1996). However, it also reduces germination. Grasses become less productive over time with too much retained material. Grazing and fire serve to temporarily reduce this thatch layer. Principles of grassland management Diverse grasslands are very resilient and in many ways are preadapted to the expected climate changes of higher temperatures, increased fire frequency, and variable precipitation (Anderson et al. 2018). Grasslands are adapted to disturbance and fluctuations in resource availability. Native grass species such as little bluestem and sideoats grama retreat quickly into dormancy when water is unavailable and revive just as quickly when moisture returns (Figure 6-3). 105 Figure 6-3. Native Prairie mix green roof in Wimberley Texas. The roof is growing in shallow media (6 inches) giving the community no access to water stored deeper in the soil column. Its position on a roof top exposes it to more extreme fluctuations in temperature than those on the ground (Simmons 2015). The image on the left shows the roof after being deprived of water for three months (April-June). The image on the right shows the community’s recovery following the application of water. Many grassland species exhibit wide moisture, temperature, and soil condition tolerances and are able to grow across a wide geographic range (Diggs 1999). These are the characteristics the Northern Institute of Applied Climate Science Adaptation workbook recommends selecting for when introducing species to improve resilience to climate change (Swanston et al. 2016). Extant native grasslands are often small; increasing their size and the connectivity between them will enhance resilience and simplify application of management strategies such as prescribed fire (Anderson et al. 2018). Reintroduction of appropriate disturbance regimes is particularly important with grasslands. Prescribed fire is an ideal tool to retard woody encroachment, periodically remove thatch, stimulate herbaceous productivity, increase species diversity, and stabilize late successional vegetation (Bowles and Jones 2013). Prescribed mowing can be used as a substitute for fire, but the effects are not entirely equivalent. Mowing will prevent woody encroachment, but it will not remove thatch or result in mortality of most woody species. As with woodlands, increasing spatial heterogeneity by using variable disturbance patches, with some on longer return interval will help build overall community and species diversity (Gordijn and O'Connor 2021). Many of the grasslands within the project area have lost significant diversity to overgrazing, cultivation, or conversion to pasture or other vegetation types. Reintroduction of natural disturbance is sometimes not enough to rebuild diversity (Novak et al. 2021). It must be paired with direct species addition through seed or live planting. Seeding is the most cost-effective method, though live plantings can be used if irrigation is available. Live plantings are typically not feasible for large areas, but have a better chance of success in moist environments such as riparian. As with woodlands, creating spatial heterogeneity within and between grassland patches will enhance overall diversity. In most cases, seeding needs to be coordinated with prescribed fire or some other treatment to reduce thatch and allow good seed contact with the soil. Additionally, sites with heavy infestations of invasive species, particularly mat forming grasses like King Ranch bluestem, will need management to begin before seeding. 106 Grassland Goal and Strategy Summary Table 9. Grassland Goals and Strategy Summary Target community Grassland - Savanna or prairie structure with <30% woody cover. Diverse species composition. Goals Develop unit-level spatial heterogeneity - patch mosaic of differing prescribed fire/mowing return intervals and species composition Identify and enhance refugia - microtopography, shaded/fire protected areas Woody cover <30%. Separation of canopy between mottes. Reduction of ladder fuels particularly at boundary with woodland Increase connectivity to other grasslands within the unit Enhance species diversity. Shift composition to: Encourage conservative grasses Encourage substantial forb cover with emphasis on wildlife value Introduce or encourage native species native to the Edwards Plateau or Texas Blackland Prairie with wide moisture and temperature tolerances or wide geographic ranges, with particular emphasis on heat tolerance. See Appendix 6. Manage invasive species. See Appendix 4. Strategies Frequent prescribed fire. Varied return interval appropriate to ecosite and condition. Primarily summer and fall, occasionally winter. Seeding in coordination with prescribed fire Mechanical thinning in coordination with prescribed fire Invasive species management Prescribed fire Prescribed fire should be used adaptively in response to fuel loads, community composition, and desired goals. Generally, a 3 to 5 year return interval will be appropriate for the communities within the project. However, spatial diversity should be encouraged with varied return intervals and changing seasons of burn, with a preference for summer or fall burns, with some patches on longer schedules. Project areas on ecological sites with thinner soils and low water availability require longer return intervals while deeper soil and higher water availability require shorter intervals because the fuel loads build more quickly and often woody encroachment is faster. Return interval, and target intensity, and season of burn should be partially driven by the community composition. Woody species become resistant to fire at different stages. Resprouting species such as mesquite are able to recover from fire soon in their life cycle, while non-resprouting species such as Ashe juniper remain sensitive to low/moderate intensity fire until they reach approximately 8 to 12 feet tall. King Ranch bluestem is strongly favored by dormant season burns (Wildflower Center, unpublished data) but often reduced by growing season burns. Growing season burns tend to reduce woody cover more significantly than dormant season burns. 107 Mechanical thinning In target grassland communities, encroaching woody species should be removed using methods that minimize soil disturbance, such as tree shears or masticators, or hand cutting with chainsaws. Ideally, slash will be left on site but compressed or shattered to a height of less than two feet. This will allow the slash to be consumed in subsequent prescribed fire. Cut slash can be used to protect seedlings. Seeding Seeding is the preferred method for species addition in grasslands. Seeding should be coordinated with prescribed burning or other treatments that allow good seed/soil contact. No-till drill seeding is the preferred method of addition, though hand seeding can be used in difficult to access locations. See woodland for discussion of seeding windows. Seed hay involves cutting hay from a healthy grassland while many species are in seed and then rolling the material out where additional diversity is desired. It can be an effective way to introduce a variety of commercially unavailable species, though invasive species can also be brought in. 6.3.3 BOTTOMLAND/RIPARIAN MANAGEMENT Riparian areas are the hydrophilic communities lining waterways and bottomlands are the low basins surrounding them. They hold more water than the surrounding uplands and often have distinctive soils. Therefore, they contain unique, hydrophilic, versions of grassland and woodlands communities. The majority of these areas within the project are currently deciduous woodlands, though some contain mesic grasslands. The communities found here, though connected to the upland communities, are subject to unique stressors, namely periodic flooding. These communities are also critically important to maintaining bank stability of the waterway, slowing and cleaning water entering it, removing sediment and pollutants from floodwater, providing shade and organic matter to in-stream habitats, and providing wildlife corridors as well as unique upland habitats. Larger waterways (perennial and intermittent) should be restored to gallery forest. Many of the strategies discussed in Woodland Management can be successfully employed in wooded riparian and bottomland areas. Increased native diversity should be encouraged invasive management and seeding or planting native woody and herbaceous species such as those listed in Appendix 6. Primary goals guiding species selection in the riparian areas are enhanced bank stability and water quality. Many species found in central and southwest Texas have been given draft stability ratings based on their contribution to bank stability (Nueces River Authority 2014). Stability ratings range from 1 to 10, with 1 approximating the bare ground and 10 anchored rock. Ideally, riparian areas will be dominated by plants with stability ratings between 6 and 9. Stability ratings of 7 or higher are considered to be the minimum for acceptable bank stability. However, combinations of species, particularly woody species in association with grasses or sedges, can provide higher stability than reflected in individual species ratings (Nueces River Authority 2014). In addition to stability ratings, USFWS wetland indicator status should be considered. Riparian areas should contain a mix of obligate wetland, facultative wetland and facultative species, dependent on water availability. Perennial waterways, generally found in the contributing zone, can support a larger complement of obligate and facultative wetland species and intermittent waterways, generally found in the recharge zone, will require a higher proportion of 108 facultative species. Regardless of the mix, it is important that all riparian areas contain some species from the facultative groups to provide stability as water availability fluctuates (S. Nelle pers. comm.). Native woody species are most likely to establish during a wet year. Most woody species volunteer aggressively in bottomland sites, so native species additions should be supported where needed. Emphasis should be on seeding unless supplemental water can be supplied. Seed can be relatively inexpensively added each year. Live staking and can be considered when sufficient subsurface moisture is consistently present to support the live stakes. This condition often occurs in and adjacent to perennial streams within the Blackland Prairie ecoregion. Low-tech grade controls and repairing existing headcuts should be considered when appropriate. 109 Bottomland goals and strategy Table 10 Bottomland Goals and Strategy 110 6.3.4 RARE AND ENDANGERED SPECIES Golden-cheeked warbler habitat Golden-cheeked warblers are federally listed as endangered. They nest only in central Texas, in ravines and canyons with woodlands of mature Ashe-juniper mixed with oak, elm, and other hardwood species. Mature Ashe junipers are trees that are at least 15 feet in height with a trunk diameter of about five inches at four feet above the ground (Campbell 2003). Woodlands that provide suitable habitat have 50- 100% canopy closure and canopy height of 20 feet or more (Campbell 2003). The golden-cheeked warbler nesting season begins in March, and they are present in Texas through July.5 Activities in potential golden-cheeked warbler habitat need to adhere to current state and national requirements for land management activities in and around endangered species habitat. These can be modified to provide greater protection to endangered species where conditions warrant. Texas golden- cheeked warbler guidelines advise maintaining at least a 300-foot-wide buffer of woodland vegetation around Golden-cheeked Warbler habitat to minimize predation (Campbell 2003). The guidelines also state that when vegetation clearing and maintenance activities near habitat are necessary, they should not occur during the March-August nesting season to avoid adverse impacts such as disturbance of nesting and feeding birds. The USFWS Golden-Cheeked Warbler Recovery Plan6 lists habitat loss as the key threat to the species. In addition to avoiding impacts to existing habitat, the Texas guidelines provide the following recommendations for habitat restoration, which could be a goal in areas where conditions are suitable. In mesic areas where small junipers (15 ft. or less) are dominant, small junipers could be thinned to improve resilience and health of remaining trees. Thinning would encourage hardwood regeneration, especially if some slash is left in place to provide protection for hardwood seedlings. If large junipers are dominant, several small openings per acre would encourage hardwood regeneration. These openings should be protected from browsing and left to regenerate naturally or planted to native hardwoods. In each of these examples, the idea is to restore areas that may once have provided habitat to the natural oak-juniper woodland capable of growing on the site (Campbell 2003). Staff should collaborate with the Balcones Canyonlands Conservation Plan Coordinating Committee Secretary and/or Balcones Canyonlands Preserve staff when planning management activities within occupied habitat that could disrupt canopy cover, such as mechanical canopy opening, and prescribed burning intended to improve woodland health. 5 https://tpwd.texas.gov/huntwild/wild/species/gcw/ 6 https://ecos.fws.gov/docs/recovery_plan/920930f.pdf 111 Black-capped vireo habitat In May 2018, the black-capped vireo was removed from the federal List of Endangered and Threatened Wildlife; however, it remains listed in Texas as vulnerable TPWD 20237. Impacts to the bird and its habitat should be avoided, and management can be tailored to improve vireo habitat. Black-capped vireo habitat consists of oak-juniper woodlands with a patchy, two-layered aspect, featuring a shrub and tree layer with open, grassy spaces. They require foliage reaching to ground level for nesting cover; and are known to return to same territory, or one nearby, year after year. Deciduous and broad-leaved shrubs and trees are required to provide insects for feeding. The nesting season is from March to late summer8. Prescribed burning can be a good tool to maintain or create the desired vegetation structure. Cool season burns (before March 15) can be used to control small juniper and maintain open shrubland. More intense fires can help create vireo habitat. Growing-season burns should be done only in areas that do not currently support black-capped vireos. Selective vegetation thinning can also be used to maintain or create vireo habitat. Good nesting habitat generally has between 30-60% shrub canopy. Selective removal of species such as juniper, mesquite and pricklypear, during the non-breeding season (September-February) can be used to keep the habitat favorable by maintaining the proper shrub canopy and encouraging growth of broad-leaved shrubs (Campbell 2003). Radical changes in shrub canopy from one year to the next should b e avoided. Grazing and browsing management should be employed because excessive browsing destroys the thick woody growth needed for nest concealment. Jollyville Plateau Salamander/Austin Blind Salamander Critical habitat for several cave and karst species occur within the project areas, and management should adhere to USFWS recommendations and existing BCP and BCCP management plans, permits and governing documents. Bracted Twistflower Bracted twistflower (Strepanthus bracteaus) is listed as threatened un the Endangered Species act, and critical habitat occurs within the project area. Bracted twistflower is a rare annual forb, native to the southeastern edge of the Edwards Plateau. It is found in oak-juniper woodlands and associated openings on slopes and in canyon bottoms with shallow, well drained gravelly clays and clay loams over limestone. These conditions are found along the rocky slopes of the Balcones Escarpment. The main threats to bracted twistflower include habitat destruction from development, browsing by white tailed dear, reduced light levels from an increased density of woody plant cover, small population sized and lack of genetic diversity9. Management should adhere to USFWS recommendations and existing BCP and BCCP management plans, permits and governing documents. 7 Texas Parks and Wildlife Department, Wildlife Division, Diversity and Habitat Assessment Programs. TPWD County Lists of Protected Species and Species of Greatest Conservation Need. [Travis County and revised 01/04.2023]. Accessed 03/30/23. 8 https://tpwd.texas.gov/gis/rtest/ 9 US Fish and Wildlife Service. Rare Central Texas Wildflower Listed as Threatened under Endangered Species Act. https://www.fws.gov/press-release/2023-04/bracted-twistflower-listed-threatened-under-endangered-species-act 112 Texas Fatmucket Texas fatmucket (Lampsilis bracteate), a freshwater mussel found in tributaries of the Colorado River, has critical habitat within the project area and is proposed endangered. The Texas fatmucket requires a host fish, the Guadalupe Bass to transform from the larva stage to a self-supporting juvenile mussel10. The species’ habitat is flowing streams and rivers of the Edwards Plateau, with substrates of stable sand, firm mud, stable sand and gravel bottoms. Management should adhere to USFWS recommendations. 6.4 PRIORITIZING IMPLEMENTATION Restoration and management are on-going processes that must be carried out in order to realize and maintain returns on investment in the form of robust ecosystem services and healthier, more resilient human communities. Thus, beginning the restoration process represents a commitment to continue. The fundamental question for the land manager is often, “If we start the process, will we be able to continue and be successful?” Implementation plans and adaptive management strategies should identify decisions points and response actions for whether the process of restoration and management cannot be continued, or if management goals must be dramatically altered, then managers. Ecological restoration and land management actions are prioritized based on a variety of considerations. Elements that must be present include: Staff, available and qualified Funding Equipment - - - - Access for equipment and staff Other considerations that affect decision-making include: - - Social vulnerability of the nearby community Environmental vulnerability or risk of negative environmental outcome o i.e. drought mortality or intense wildfire - Density and proximity of neighboring structures - Regional conservation context o i.e. the significance of the site to provision of ecosystem services at a local or regional scale - Restoration potential of the site - Cost or resource input, per acre - Codependence of multiple of strategies. year 5, and monitor after each. - Project complexity o E.g. Must thin in year 1, burn in year 3, seed in year 4, invasive species management in o Number and interdependence of resources and actions o Dependence on resources not under the land manager’s direct administrative control 10 US Fish and Wildlife service. https://www.fws.gov/species/texas-fatmucket-lampsilis-bracteata 113 o Required community outreach or coordination o Restricted timelines for implementation o Need for and difficultly of public access management - Potential for maintaining restoration investment (not regressing to the pre-treatment state or to a different undesirable state) with continued management over time - Community (human) support or resistance o Expressed support or resistance o Potential for perceived negative outcomes leading to expressed dissatisfaction o Volunteer availability - Restrictions to operations o E.g. Endangered species habitat, critical environmental features, cultural resources, narrow seasonality or weather windows for operations - Permitting o E.g. Prescribed burns, endangered species habitat - Training and licensing o E.g. Pesticide application, wildland fire - Park development plans - Current and future adjacent land uses. o E.g. Adjacent development (roads, homes, schools, businesses) or conservation lands. 114 7 Monitoring & Adaptive Management Monitoring performance quantitatively and qualitatively is an essential part of the land management practice. Monitoring is important for both staff and the public. Information gathered allows staff to communicate the benefits of restoring ecosystem function in urban areas as well as inform adaptive management decisions. Additionally, monitoring can support the larger sustainability initiatives and programs occurring within the City of Austin and beyond. It is anticipated that there will be long-term and short-term monitoring projects. Long-term projects will be repeated annually or bi-annually depending upon the methodology and will be administered over multiple years. The goal of this type of monitoring project is to examine the long-term effects of management and ecosystem performance. A short-term monitoring project would be administered to quickly understand a question that needs more data to address design or management elements. For instance, looking at different herbicides on a specific species during a growing season might be a possible short-term monitoring project. It is important that monitoring first inform PARD and BCP staff in achieving primary management goals. However, it is also important to consider the larger regional needs and questions regarding restoration/land management and how Austin’s work can dovetail or support the community of practice and other monitoring projects. Working with other organizations to develop monitoring methodologies is beneficial because it reduces protocol development, increases the data’s potential impact, and becomes part of a larger study and story. The following suggested metrics are a starting point. High-level metrics include patch size, habitat connectivity, flood risk reduction. The conservation-based metrics are biodiversity, habitat connectivity, habitat quality, stormwater capture, and heat island effect reduction. 7.1 MONITORING PARAMETERS 7.1.1 POTENTIAL FIRE BEHAVIOR Monitoring and mapping of fuel models over time should reflect how crown fire probability or other important fire behavior metrics, such as flame length or fire intensity, change in response to management activities. This will be important for verifying and communicating the effect of management activities and their associated costs on wildfire risk. 7.1.2 VULNERABILITY INDICES Monitoring and mapping of the components of the Environmental Vulnerability Index that can be affected by management – components such as crown fire probability or other fire behavior metrics as described above, as well as elements of Vegetation Community Condition as described below – will allow these indices to be updated in response to management. This will be important for verifying and communicating the effect of management activities and their associated costs on vulnerability to climate-driven events including wildfire. 115 7.1.3 BIODIVERSITY AND HABITAT QUALITY Biodiversity metrics are integral to vulnerability indices and help inform progress toward regional-level initiatives to protect our natural heritage and conserve rare and endangered species and vegetative communities. Parameters such as herbaceous cover, canopy cover, species composition, and woody stem density are important for assessing change in plant communities over time with respect to management goals. Vegetation monitoring may be short term (e.g. before or after a discrete management project) or long-term (e.g. conducted along permanent monitoring locations in large management units) depending on the question being addressed. Where possible, vegetation monitoring protocols should produce data that can be pooled with data from other entities such as the BCP or WQPL and analyzed via meta-analyses. Elements of bird or pollinator communities are also valuable as these taxa are often attractions to park users for wildlife viewing, enhance the aesthetic and mental health benefits provided by natural areas, and relate to other City of Austin conservation goals and certifications such as the Mayor’s Monarch Pledge, Austin’s Community Wildlife Habitat Certification, Austin’s Bee City certification, and Austin’s Bird City certification. Monitoring additional wildlife taxa is something the program can grow into with more staff and partnerships. PARD is part of the Urban Wildlife Information Network and analysis of the data collected on PARD lands would be a useful starting point. Habitat Quality is an important part of assessing ecological function. The parameters used to assess conditions for this report can be used as a starting point for creating a Habitat Quality Index. Collaboration with other organizations and stakeholders is recommended to coordinate the collection of highest priority data and organization and distribution of the data. A Nature Conservancy Biodiversity and Ecosystem Monitoring program conducted in Austin (Belaire et al. 2017) provides a possible model. 7.1.4 HABITAT CONNECTIVITY Connectivity affects the capacity of the landscape to facilitate movement of species, resources, and propagules between larger habitat patches as well as the ability of parklands to provide immersive experiences for park users. Connectivity supports migration and allows some species to effectively increase their habitat area. For example, most wild bees need a patch size of 48 to 198 acres to fully support a population. However, much smaller patches are valuable as long as they are close enough that the bees can move between them, using patches as steppingstones. 7.1.5 HYDROLOGIC FUNCTION Hydrologic functions such as stormwater capture and stream channel stability can be important indicators of ecosystem functions or disturbances occurring higher in the watershed. The stormwater capture metric is about monitoring the site's capacity to slow, hold, and infiltrate water. Stream channel stability and erosion is also a concern in urbanized areas, particularly under projected climate change scenarios. Significant stream erosion events such as along Shoal Creek and Country Club Creek in Roy G. Guerrero Park have degraded parkland and threatened critical infrastructure as well as homes and businesses. PARD will continue to work with entities such as Watershed Protection Department to facilitate this monitoring. 116 7.2 ADAPTIVE MANAGEMENT Management that evolves with new information and changing conditions is called adaptive management, a decision-making process in which management is informed by monitoring. Strategies for achieving management goals should result from and change throughout a process of discovery involving formal experimentation and/or an evolution of management strategies over time as the site, its management needs, and the context within which the site is situated change. Timely analysis of the information collected via a well-designed monitoring program is how managers evaluate progress toward management goals. The steps of adaptive management are: 1. Clarify the project mission, goals, and objectives. 2. Develop the monitoring and management plans based on the best available research and other information. 3. Begin implementing the monitoring plan to gather baseline data. 4. Begin implementing the management plan to initiate environmental change. Continue implementing the monitoring plan to gather information about the change occurring in the system. 5. Analyze monitoring data and evaluate the effectiveness of management actions relative to the project goals and objectives. 6. Adapt the management plan to improve effectiveness. Continue to update and augment the 7. management plan with new research and other information. Implement the new iteration of the management plan. Continue implementing the monitoring plan. Carry on the cycle of implement, monitor, evaluate, adapt. Assessment parameters should be selected based on the goals for the site, practicality of implementation and availability of funding. Selected parameters should provide insight into the baseline condition of the site and the impact of management action or inaction. Monitoring will need to be done at several spatial and temporal scales to be meaningful. Ideally, monitoring activities should include both rapid visual assessments that can be done frequently over a large area and less frequent, but higher resolution studies. Actions are prescribed at the unit or community level, which requires targeting appropriate strategies for local threats and conditions as described in 6.2. The potential management actions can be organized by the seven land management goals. 1. Engage partners and community representatives in stewardship 2. Sustain Ecological Functions 3. Reduce Impact of Biological Stressors 4. Reduce risk & long-term impacts of severe disturbance 5. Maintain & Enhance species and structural diversity 6. Promote Connectivity 7. Facilitate Community adjustment to anticipated change 117 7.3 NEXT STEPS/POLICY INTERSECTIONS - Pursue staffing, equipment, budget, and workspace needs through the City budget process or grants where possible. - Create and implement workplans according to the criteria in the Prioritizing Implementation section. Where community stakeholders are currently active or can be recruited, collaboratively develop and implement workplans to allow for unified management of project sites. - Design and begin implementation of a core monitoring program focused on the priorities identified in [Section 7.1]. - Consider using geospatial analytical tools such as ArcGIS, Flammap, or Farsite to model the response of fire behavior and fire risk to potential land treatments to assist with prioritization and project planning. For example, given a finite project budget of, $50,000, what would be the effect of various land treatments – shaded fuel breaks, selective thinning, dripline treatments, invasive species removal, etc – on fire behavior and fire risk to values such as PARD land capital assets or adjacent homes? Also consider using these tools to model the effect of implemented projects to communicate to land managers, executives, elected officials, and the public fire risk reduction benefits per dollar spent. - Continually update and improve site assessments to incorporate new data or changing conditions. This information may be gathered via formalized monitoring, informal observational data gathered during development of work plans, or from outside sources such as third-party researchers. Identify reference sites and potential refugia - 118 8 References Adams, M. A. 2013. Mega-fires, tipping points and ecosystem services: Managing forests and woodlands in an uncertain future. Forest Ecology and Management 294:250-261. Abrams, M. D. 1992. Fire and the Development of Oak Forests. Bioscience 42:346-353 Amthor, J. S., V. H. Dale, N. T. Edwards, C. T. Garten, C. A. Gunderson, P. J. Hanson, M. A. Huston, A. W. King, R. J. Luxmoore, S. B. McLaughlin, G. Marland, P. J. Mulholland, R. J. Norby, E. G. O’Neill, R. V. O’Neill, D. S. W. M. Post, D. Shriner, E. Todd, T. J. Tschaplinski, R. S. Turner, G. A. Tuskan, and S. D. Wullschleger. 1998. Terrestrial Ecosystem Responses to Global Change: a research strategy. Oak Ridge National Laboratory, Oak Ridge, Tennessee. Anderegg, W.R., Konings, A.G., Trugman, A.T., Yu, K., Bowling, D.R., Gabbitas, R., Karp, D.S., Pacala, S., Sperry, J.S., Sulman, B.N. and Zenes, N., 2018. Hydraulic diversity of forests regulates ecosystem resilience during drought. Nature, 561(7724), pp.538-541. Anderson H.E. 1982 Aids to Determining fuel models for estimating fire behavior. United States Department of Agriculture, Forest Service. General Technical Report INT-122 Anderson, M. G., M. A. Ahlering, M. M. Clark, K. R. Hall, A. O. Sheldon, J. Platt, and J. Prince. 2018. Resilient Sites for Terrestrial Conservation in the Great Plains Region. The Nature Conservancy, Eastern Conservation Science and North America Region. Andruk, C.M., Schwope, C. and Fowler, N.L., 2014. The joint effects of fire and herbivory on hardwood regeneration in central Texas woodlands. Forest Ecology and Management, 334, pp.193-200. Archer, S. R., E. M. Andersen, K. I. Predick, S. Schwinning, R. J. Steidl, and S. R. Woods. 2017.Woody plant encroachment: Causes and consequences. Pages 25-84 in D. D. Briske, editor. Rangeland Systems. Springer, Cham, Cham, Switzerland. Austin Parks Foundation (APF) and Trust for Public Land. 2019. Healthy Parks Plan for Travis, Bastrop, and Caldwell Counties. Beck, H. E., N. E. Zimmerman, T. R. McVicar, N. Vergopolan, A. Berg, and E. F. Wood. 2018. Present and future Köppen-Geiger climate classification maps at 1-km resolution. Scientific Data 5. Belaire, J. A., C. Higgins, N. Folwer, T. H. Keitt, and S. Jha. 2018. Waller Creek biodiversity and ecosystem monitoirng project. Spring and Summer 2018 update. The Nature Conservancy, Austin. Bertelsen, M., S. Windhager, and M. Simmons. 2010. Recommended Land Management for the Water Quality Protection Lands. Submitted to Austin Water Utility Wildland Conservation Division. Birdsey, R. A., A. J. Dugan, S. P. Healey, K. Dante-Wood, F. Zhang, G. Mo, J. M. Chen, A. J. R. Hernandez, Crystal L., and J. McCarter. 2019. Assessment of the influence of disturbance, managemet activities, and environmental factors on carbon stocks of the U.S. national forests. U.S Department of Agriculture - Forest Service - Rocky Mountain Research Station,. Berryman, E., M. Battaglia, and C. Hoffman. 2015. Fire effects for different slash management techniques in lower montane ponderosa pine forests. Boulder County Parks and Open Space Department, Boulder, CO. Bixler, R. P. and E. Yang. 2020. Climate Vulnerability in Austin: A multi-risk assessment. An Austin Area Sustainability Indicators and Planet Texas 2050, Unpublished Technical Report. Bottero, A., D'Amato, A.W., Palik, B.J., Bradford, J.B., Fraver, S., Battaglia, M.A. and Asherin, L.A., 2017. Density‐dependent vulnerability of forest ecosystems to drought. Journal of Applied Ecology, 54(6), pp.1605-1614. 119 Bowles, M. L., and M. D. Jones. 2013. Repeated burning of eastern tallgrass prairie increases richness and diversity, stabilizing late successional vegetation. Ecological Applications 23:464-478. Bradley, B. A., D. M. Blumenthal, D. S. Wilcove, and L. H. Ziska. 2010. Predicting plant invasions in an era of global change. Trends in Ecology and Evolution 25:310-318. Braga, A.A., and B.J. Bond. 2008. Policing Crime and Disorder Hot Spots: A Randomized Controlled Trial. Criminology 46, 3:577-607. Brandt, L. A., Rottler, C., Gordon, W., Clark, S. L., O’Donnell, L., Rose, A.., Rutledge, A., & King, E. 2020. Vulnerability of Austin’s urban forest and natural areas: a report from the Urban Forestry Climate Change Response Framework. Report NFCH-5. Houghton, MI: U.S. Department of Agriculture, Climate Hubs. 83 p. Bray, W. L. 1904. The Timber of the Edwards Plateau of Texas; Its Relations to Climate, Water Supply and Soil. U.S. Department of Agriculture, Bureau of Forestry. Brunner, E. 1997. Stress and the Biology of Inequality. British Medical Journal 314: 1472-76. Bush, J. K., and O. W. Van Auken. 2018. Comparison of mortality of Quercus stellata (post oak) after a Central Texas drought. Phytologia 100:167-176. Campbell, L. 2003. Endangered and threatened animals of Texas: their life history and management in W. Division, editor. Texas Parks and Wildlife Department, Austin, Texas.Chalfont, G.E., and S. Rodiek. 2005. Building Edge: An Ecological Approach to Research and Design of Environments for People with Dementia. Alzheimer's Care Today 6, 4: 341. Chen, D., X. Wang, M. Thatcher, G. Barnett, A. Kachenko, and R. Prince. 2014. Urban Vegetation for Reducing Heat Related Mortality. Environmental Pollution 192: 275-284. Choat, B., Brodribb, T.J., Brodersen, C.R., Duursma, R.A., López, R. and Medlyn, B.E., 2018. Triggers of tree mortality under drought. Nature, 558(7711), pp.531-539. City of Austin, 2020. City of Austin Climate Equity Plan, prepared by Austin Climate Equity Plan Steering Committee. Collins, O. B., F. E. Smeins, and D. H. Riskind. 1975. Plant Communities of the Blackland Prairie of Texas. Pages Pages 75-88 in Prairie: a Multiple View; Fourth North American Prairie Conference. University of North Dakota Press, Grand Forks, North Dakota. Colcombe, S., and A.F. Kramer. 2003. Fitness Effects on the Cognitive Function of Older Adults: A Meta- Analytic Study. Psychological Science 14, 2: 125-130. Crompton, J.L. 2001. Parks and Economic Development. PAS Report No. 502. American Planning Association, Chicago, Illinois. Crouchet, S. H., J. Jensen, B. F. Schwartz, and S. Schwinning. 2019. Tree mortality after a hot drought: Distinguishing density-dependent and -independent drivers and why it matters. Frontiers in Forests and Global Change 2:1-14. Diamond, D. D., G. A. Rowell, and D. P. Keddy-Hector. 1995. Conservation of Ashe Juniper (Juniperus ashei Buchholz) Woodlands of the Central Texas Hill Country. Natural Areas Journal 15:189-197. Diamond, D. D., and F. E. Smeins. 1985. Composition, classification and species response patterns of remnant tallgrass prairies in Texas. American Midland Naturalist 113:294-308. Diamond, D. D., and F. E. Smeins. 1993. The Native Plant Communities of the Blackland Prairie.in S. M. R. and and J. C. Yelderman Jr., editors. The Texas Blackland Prairie: Land, History & Culture. Baylor University Press, Waco, Texas. 120 Diamond, D. D., and C. D. True. 2008. Distribution of Junperus woodlands in central Texas in relation to general abiotic site type.in O. W. Van Auken, editor. Western North American Juniperus communities: a dynamic vegetation type. Springer, New York, New York, USA. Diggs, G. M. 1999. Shinners & Mahler's Illustrated Flora of North Central Texas. Botanical Research Institute of Texas, Fort Worth, Texas. Dorman, M., Svoray, T., Perevolotsky, A., Moshe, Y. and Sarris, D., 2015. What determines tree mortality in dry environments? a multi‐perspective approach. Ecological Applications, 25(4), pp.1054- 1071. Dunbar, J.A., Allen, P.M. and Bennett, S.J., 2010. Effect of multiyear drought on upland sediment yield and subsequent impacts on flood control reservoir storage. Water Resources Research, 46(5). Dunkerley, D.L. 2000. Assessing the influence of shrubs and their interspaces on enhancing infiltration in an arid Australian shrubland. Range. J. 22:58–71. Duvall, J., and R. Kaplan. 2013. Exploring the Benefits of Outdoor Experiences on Veterans. Report Prepared for Sierra Club Military Families and Veterans Initiative. Report prepared for the Sierra Club Military Families and Veterans Initiative. Dyksterhius, E. J. 1946. The Vegetation of the Fort Worth Prairie. Ecological Monographs 16:1-29. Fan, Y., K.V. Das, and Q. Chen. 2011. Neighborhood Green, Social Support, Physical Activity, and Stress: Assessing the Cumulative Impact. Health & Place 17, 6:1202-211. Fedrowitz, K., J. Koricheva, S. C. Baker, D. B. Lindenmayer, B. Palik, R. Rosenvald, W. Beese, J. F. Franklin, J. Kouki, E. Macdonald, C. Messier, A. Sverdrup-Thygeson, and L. Gustafsson. 2014. REVIEW: Can retention forestry help conserve biodiversity? A meta-analysis. Journal of Applied Ecology 51:1669-1679. Fisher, B., and J.L. Nasar. 1995. Fear Spots in Relation to Microlevel Physical Cues: Exploring the Overlooked. Journal of Research in Crime and Delinquency 32:214-239. Fisher, B.S., and J.L. Nasar. 1992. Fear of Crime in Relation to Three Exterior Site Features: Prospect, Refuge, and Escape. Environment and Behavior 24, 1:35-65. Fitzpatrick, M. C., and R. R. Dunn. 2019. Contemporary climatic analogs for 540 North American urban areas in the late 21st century. Nature Communications 10:1-7. Flanagan, B.E., Gregory, E.W., Hallisey, E.J., Heitgerd, J.L. and Lewis, B., 2011. A social vulnerability index for disaster management. Journal of homeland security and emergency management, 8(1). Fuller, R.A., K.N. Irvine, P. Devine-Wright, P.H. Warren, and K.J. Gaston. 2007. Psychological Benefits of Greenspace Increase with Biodiversity. Biology Letters 3, 4: 390-94. Gee, J. P., and M. C. Campbell. 1990. Mokan Prairie Survey. Nature Preserves System, Heritage and Conservation Program. Heritage and Conservation Program - Parks and Recreation Department - City of Austin,, Austin, Texas. Gelkopf, M., I. Hasson-Ohayon, M, Bikman, and S. Kravetz. 2013. Nature Adventure Rehabilitation for Combat-Related Posttraumatic Chronic Stress Disorder: A Randomized Control Trial. Psychiatry Research 209:485-493. Geroy, I.J., Gribb, M.M., Marshall, H.P., Chandler, D.G., Benner, S.G. and McNamara, J.P., 2011. Aspect influences on soil water retention and storage. Hydrological Processes, 25(25), pp.3836-3842. Gobster, P.H., and L.M. Westphal. 2004. The Human Dimensions of Urban Greenways: Planning for Recreation and Related Experiences. Landscape and Urban Planning 68:147–165. Gordijn, P. J., and T. G. O'Connor. 2021. Multidecadal effects of fire in a grassland biodiversity hotspot: Does pyrodiversity enhance plant diversity? Ecol Appl 31:e02391. 121 Greenberg, C. H., and B. Collins. 2021. Fire Ecology and Management: Past Present, and Future of US Forested Ecosystems. Greenwood, S., Ruiz‐Benito, P., Martínez‐Vilalta, J., Lloret, F., Kitzberger, T., Allen, C.D., Fensham, R., Laughlin, D.C., Kattge, J., Bönisch, G. and Kraft, N.J., 2017. Tree mortality across biomes is promoted by drought intensity, lower wood density and higher specific leaf area. Ecology letters, 20(4), pp.539-553. Griffith, G., S. Bryce, J. Omernik, and A. Rogers. 2007. Ecoregions of Texas. Texas Commission on Environmental Quality. Hammer, T.R., R.E. Coughlin, and E.T. Horn. 1974. The Effect of a Large Park on Real Estate Value. Journal of the American Institute of Planners 40:274-277. Hanselka, C. W., W. T. Hamilton, and B. S. Rector. 1999. Integrated Brush Management Systems for Texas. L-5164, Texas Agricultural Extension Service, College Station, Texas. Hanson, P.J. and Weltzin, J.F., 2000. Drought disturbance from climate change: response of United States forests. Science of the total environment, 262(3), pp.205-220. Hauru, K., S. Lehvävirta, K. Korpela, and D.J. Kotze. 2012. Closure of View to the Urban Matrix Has Positive Effects on Perceived Restorativeness in Urban Forests in Helsinki, Finland. Landscape and Urban Planning 107:361-69. Hayhoe, K. 2014. Climate change projections for the City of Austin. Prepared Report for City. Heerwagen, J.H., and G.H. Orians. 2002. The ecological world of children. In: Kahn, P.H.J., and S.R. Kellert (eds.), Children and Nature: Psychological, Sociocultural, and Evolutionary Investigations. MIT Press, Cambridge MA, pp. 29-64. Hegewisch, K. C., Abatzoglou, J. T., Chedwiggen, O., & Nijssen, B. (2019). Climate Mapper web tool. Retrieved October, 2019 from https:// climatetoolbox.org/tool/climate-mapper Hester, J.W., Thurow, T.L., and Taylor, C.A. 1997. Hydrologic characteristics of vegetation types as affected by prescribed burning. J. Range. Manag. 50:199–204. Hicks, R. A. and W. A. Dugas. 1998. Estimating ashe juniper leaf area from tree and stem characteristics. Journal of Range Management 51:633-637. Hondula, D.M., and A.G. Barnett. 2014. Heat-Related Morbidity in Brisbane, Australia: Spatial variation and Area-Level Predictors. Environmental Health Perspectives 122, 8: 831-836. Hyer, L., S. Boyd, R. Scurfield, D. Smith, and J. Burkel. 1996. Effects of Outward Bound Experience as an Adjunct to Inpatient PTSD Treatment of War Veterans. Journal of Clinical Psychology 52, 3:263- 278. Isbell, F., Craven, D., Connolly, J., Loreau, M., Schmid, B., Beierkuhnlein, C., Bezemer, T.M., Bonin, C., Bruelheide, H., De Luca, E. and Ebeling, A., 2015. Biodiversity increases the resistance of ecosystem productivity to climate extremes. Nature, 526(7574), pp.574-577. Jackson, R., L. Moore, W. Hoffmann, W. Pockman, and C. Linder. 1999. Ecosystem rooting depth determined with caves and DNA. Proceedings of the National Academy of Sciences 96:11387- 11392. Jessup, K. E., P. W. Barnes, and T. W. Boutton. 2003. Vegetation Dynamics in a Quercus-Juniperus Savanna: An Isotopic Assessment. Journal of Vegetation Science 14:841-852. Jiang, X., and Yang, Z.L. 2012. Projected changes of temperature and precipitation in Texas from downscaled global climate models. Climate Research 53:229-244. Johnson, D. M., J. C. Domec, Z. C. Berry, A. M. Schwantes, K. A. McCulloh, D. R. Woodruff, H. W. Polley, R. Wortemann, J. J. Swenson, D. Scott Mackay, N. G. McDowell, and R. B. Jackson. 2018. Co‐ 122 occurring woody species have diverse hydraulic strategies and mortality rates during an extreme drought. Plant, cell & environment 41:576-588. Johnston, J. D., J. H. Olszewski, B. A. Miller, M. R. Schmidt, M. J. Vernon, and L. M. Ellsworth. 2021. Mechanical thinning without prescribed fire moderates wildfire behavior in an Eastern Oregon, USA ponderosa pine forest. Forest Ecology and Management 501. Jones, J., D. Ellison, S. Ferraz, A. Lara, X, Wei, and Z Zhang. 2022. Forest restoration and hydrology. Kahn Jr., P.H., and S.R. Kellert. 2002. Children and Nature: Psychological, Sociocultural, and Evolutionary Forest Ecology and Management. 520. Investigations. MIT Press, Cambridge MA. Kaplan, R., and J.F. Talbot. 1988. Ethnicity and Preference for Natural Settings: A Review and Recent Findings. Landscape and Urban Planning 15:107-117. Kaplan, R., and S. Kaplan. 1989. The Experience of Nature: A Psychological Perspective. New York, Kaplan, S. 1995. The Restorative Benefits of Nature: Toward An Integrative Framework. Journal of Cambridge University Press. Environmental Psychology 15, 3:169-182. Kerbo, R. 1998. National Park Service Cave and Karst Program. NPS Geologic Resources Division. Kirkby, M. 1989. Nature as refuge in children’s environments. Children’s Environments Quarterly 6:7-12. Kuo, F.E., and W.C. Sullivan. 2001. Environment and Crime in the Inner City: Does Vegetation Reduce Crime? Environment and Behavior 33, 3:343-367. online summary Kuo, F.E., M. Bacaicoa, and W.C. Sullivan. 1998. Transforming Inner-City Landscapes: Trees, Sense of Safety, and Preference. Environment and Behavior 30:28-59. Knapp, A. K., and Seastedt. 1986. Detritus accumulation limits productivity of tall grass prairie. Bioscience 36:662-668. Li, Q., M. Kobayashi, H. Inagaki, Y. Hirata, Y.J. Li, K. Hirata, T. Shimizu, H. Suzuki, M. Katsumata, Y. Wakayama, T. Kawada, T. Ohira, N. Matsui, and T. Kagawa. 2010. A Day Trip to a Forest Park Increases Human Natural Killer Activity and the Expression of Anti-cancer Proteins in Male Subjects. Journal of Biological Regulators and Homeostatic Agents 24, 2:157-166. Liu, Z., M. C. Wimberly, A. Lamsal, T. L. Sohl, and T. J. Hawbaker. 2015. Climate change and wildfire risk in an expanding wildland–urban interface: A case study from the Colorado Front Range Corridor. Landscape ecology 30:1943-1957. Luttik, J. 2000. The Value of Trees, Water and Open Space as Reflected by House Prices in the Netherlands. Landscape and Urban Planning 48:161-167. Lyons, J., et al. (2000). "Grass versus trees: Managing riparian areas to benefit sterams of central North America." Journal of the American Water Resrouces Association 36(4): 919-930. Maas, J., R.A. Verheij, P.P. Groenewegen, S. de Vries, and P. Spreeuwenberg. 2006. Green Space, Urbanity, and Health: How Strong is the Relation? Journal of Epidemiology and Community Health 60:587–592. McCaw, W.M., Grobert, D.M., Brown, S.B., Strickland, S., Thompson, G.A., Gillman, G., Ball, L.M. and Robinson, C.D., 2018. Seasonal patterns and drivers of Ashe juniper foliar live fuel moisture and relevance to fire planning. Fire Ecology, 14, pp.50-64. McDowell, N. G., and C. D. Allen. 2015. Darcy's law predicts widespread forest mortality under climate warming. Nature Climate Change 5:669. McKinley, D. C., M. G. Ryan, R. A. Birdsey, C. P. Giardina, M. E. Harmon, L. S. Heath, R. A. Houghton, R. B. Jackson, J. F. Morrison, B. Murray, D. E. Pataki, and K. E. Skog. 2011. A synthesis of current 123 knowledge on forests and carbon storage in the United States. Ecological Applications 21:1902- 1924. Meinzer, F. C., Woodruff, D. R., Marias, D. E., Smith, D. D., McCulloh, K. A., Howard, A. R., & Magedman, A. L. (2016). Mapping ‘hydroscapes’ along the iso‐to anisohydric continuum of stomatal regulation of plant water status. Ecology Letters, 19, 1343–1352. Mitchell, R., and F. Popham. 2008. Effect of Exposure to Natural Environment on Health Inequalities: An Observational Population Study. The Lancet 372: 1655-1660. Mitchell, R., T. Astell-Burt, and E.A. Richardson. 2011. A Comparison of Green Space Indicators for Epidemiological Research. Journal of Epidemiology and Community Health 65, 10:853-58. Montgomery, D. R. (1997). "What's best on the banks?" Nature 388(6640): 328-329. Mooney, P., and P.L. Nicell. 1992. The Importance of Exterior Environment for Alzheimer Residents: Effective Care and Risk Management. Healthcare Management Forum 5, 2: 23-29. Moore, G. W., C. B. Edgar, J. G. Vogel, R. A. Washington‐Allen, R. G. March, and R. Zehnder. 2016. Tree mortality from an exceptional drought spanning mesic to semiarid ecoregions. Ecological Applications 26:602-611. More, T.A., T.H. Stevens, and P.G. Allen. 1988. Valuation of Urban Parks. Landscape and Urban Planning 15:139-152. Morita, E., S. Fukuda, J. Nagano, et al. 2007. Psychological Effects of Forest Environments on Healthy Adults: Shinrin-Yoku (Forest-Air Bathing, Walking) As a Possible Method of Stress Reduction. Public Health 121, 1:54-63. Murphy, P.C., Knowles, J.F., Moore, D.J., Anchukaitis, K., Potts, D.L. and Barron-Gafford, G.A., 2020. Topography influences species-specific patterns of seasonal primary productivity in a semiarid montane forest. Tree Physiology, 40(10), pp.1343-1354. Murray, D. B., and J. D. White. 2013. Loss of Neighbors, Fire, and Climate Effects on Texas Red Oak Growth in a Juniper-dominated Woodland Ecosystem. The American Midland Naturalist 170:348-369, 322. Murray, D. B., et al. (2013). "Woody vegetation persistence and disturbance in central Texas grasslands inferred from multidecadal historical aerial photographs." Rangeland Ecology & Management 66(3): 297-304. Nasar, J.L., and K. Jones, K. 1997. Landscapes of Fear and Stress. Environment and Behavior 29:291-323. Nasar, J.L., B. Fisher, and M. Grannis. 1993. Proximate Physical Cues to Fear of Crime. Landscape and Urban Planning 26:161-176. Nelle, S. 2009a. Common plants of riparian areas--Central--Southwest Texas with wetland indicator (WI) and proposed stability rating (SR). Natural Resources Conservation Service, San Angelo, TX. Contact: steve.nelle@tx.usda.gov. Nordt, L. C., T. W. Boutton, C. T. Hallmark, and M. R. Waters. 1994. Late Quaternary vegetation and climate changes in central Texas based on the isotopic composition of organic carbon. Quaternary Research 41:109-120. Nowacki, G. J., and M. D. Abrams. 2008. The Demise of Fire and “Mesophication” of Forests in the Eastern United States. Bioscience 58:123-138. Nowak D.J., D.E. Crane, and J.C. Stevens. 2006. Air Pollution Removal by Urban Trees and Shrubs in the United States. Urban Forestry & Urban Greening 4: 115–23. Nowak, D.J., S.M. Stein, P.B. Randler, E.J. Greenfield, S.J. Comas, M.A. Carr, and R.J. Alig. 2010. Sustaining America's Urban Trees and Forests: A Forests on the Edge Report. U.S.D.A. Forest 124 Service, General Technical Report NRS-62. Newtown Square, PA: Northern Research Station, 27 pp. Novak, E. N., M. Bertelsen, D. Davis, D. M. Grobert, K. G. Lyons, J. P. Martina, W. M. McCaw, M. O'Toole, and J. W. Veldman. 2021. Season of prescribed fire determines grassland restoration outcomes after fire exclusion and overgrazing. Ecosphere 12. Nueces River Authority. 2014. Your Remarkable Riparian. Nueces River Authority. O'Donnell, L., B. J. Pickles, C. M. Campbell, L. L. Moulton, N. M. Hauwert, and M. A. Gorzelak. 2020. Native tree and shrub canopy facilitates oak seedling regeneration in semiarid woodland. Ecosphere 11. O'Hara, K., and B. Ramage. 2013. Silviculture in an uncertain world: Utilizing multi-aged management systems to integrate disturbance. Forestry 86. Olinde, L., S. Lane, A. Richter, A. González, and M. Scoggins. 2021. Stream corridors in the Blackland Prairie, City of Austin Watershed Protection Department, RR-21-01 O’Rourke, P.A., and W.H. Terjung. 1981. Urban parks, energy budgets and surface temperatures. Archives for Meteorology, Geophysics, and Bioclimatology 29, 4: 327-344. Owens, M. K. and R. K. Lyons. 2002. Evaporation and interception water loss from Juniper communities on the Edwards Aquifer Recharge Area. UREC-02-028, Texas A&M Agricultural Research & Extension Center at Uvalde, Uvalde, TX. Owens, M. K., R. K. Lyons, and C. L. Alejandro. 2006. Rainfall partitioning within semiarid juniper communities: effects of event size and canopy cover. Hydrological Processes 20:3179-3189. Paquet, C., T.P. Orschulok, N.T. Coffee, N.J. Howard, G. Hugo, A.W. Taylor, R.J. Adams, and M. Daniel. 2013. Are Accessibility and Characteristics of Public Open Spaces Associated with a Better Cardiometabolic Health? Landscape and Urban Planning 118:70-78. Park, B.J., Y. Tsunetsugu, T. Kasetani, T. Kagawa, and Y. Miyazaki. 2010. The Physiological Effects of Shinrin-yoku (Taking in the Forest Atmosphere or Forest Bathing): Evidence From Field Experiments in 24 Forests Across Japan. Environmental Health and Preventive Medicine 15, 1:18-26. Polley, H. W., D. M. Johnson, and R. B. Jackson. 2016. Canopy foliation and area as predictors of mortality risk from episodic drought for individual trees of Ashe juniper. Plant Ecology 217:1105- 1114. Polley, H. W., D. M. Johnson, and R. B. Jackson. 2018. Projected drought effects on the demography of Ashe juniper populations inferred from remote measurements of tree canopies. Plant Ecology 219:1259-1267. Ponisio, L. C., K. Wilkin, L. K. M'Gonigle, K. Kulhanek, L. Cook, R. Thorp, T. Griswold, and C. Kremen. 2016. Pyrodiversity begets plant-pollinator community diversity. Global change biology 22:1794-1808. Popham, F., and R. Mitchell. 2007. Relation of Employment Status to Socioeconomic Position and Physical Activity Types. Preventative Medicine 45: 182-88. Pretty, J., R. Hine, and J. Peacock. 2006. Green Exercise: The Benefits of Activities in Green Places. Biologist 53, 3: 143-48. Radeloff, V. C., D. P. Helmers, H. A. Kramer, M. H. Mockrin, P. M. Alexandre, A. Bar- Massada, V. Butsic, T. J. Hawbaker, S. Martinuzzi, and A. D. Syphard. 2018. Rapid growth of the US wildland-urban interface raises wildfire risk. Proceedings of the National Academy of Sciences 115:3314-3319. Reemts, C. M., W. M. McCaw, T. A. Greene, and M. T. Simmons. 2019. Short-Term Control of an Invasive C4 Grass With Late-Summer Fire. Rangeland Ecology & Management 72:182-188. 125 Reidy, J. L., F. R. Thompson, C. Schwope, S. Rowin, and J. M. Mueller. 2016. Effects of prescribed fire on fuels, vegetation, and Golden-cheeked Warbler (Setophaga chrysoparia) demographics in Texas juniper-oak woodlands. Forest Ecology and Management 376:96-106. Riskind, D. H., and O. B. Collins. 1975. The Blackland Prairie of Texas: Conservation of Representative Climax Remnants. Pages 361-367 in Prairie: a Multiple View; Fourth North American Prairie Conference. University of North Dakota Press, Grand Forks, North Dakota. Roe, J.J., C.W. Thompson, P.A. Aspinall, M.J. Brewer, E.I. Duff, D. Miller, R. Mitchell, and A. Clow. 2013. Green Space and Stress: Evidence From Cortisol Measures in Deprived Urban Communities. International Journal of Environmental Research and Public Health 10, 9: 4086-4103. Runkle, J., K. Kunkel, J. Nielsen-Gammon, R. Frankson, S. Champion, B. Stewart, L. Romolo, and W. Sweet. 2017. Texas State Climate Summary. Russell, F. L. and N. L. Fowler (2002). "Failure of adult recruitment in Quercus buckleyi populations on the eastern Edwards Plateau, Texas." The American Midland Naturalist 148(2): 201-217 Russell, B. and J. Jenkins. 2001. Review of Geology and Karst Sections of the Management Plan for the Water Quality Protection Lands, Travis County, Texas. Texas Cave Management Association, Austin, Texas. Sade, N., A. Gebremedhin, and M. Moshelion. 2012. Risk-taking plants: anisohydric behavior as a stress- resistance trait. Plant signaling & behavior 7:767-770. Sample, M., A. E. Thode, C. Peterson, M. R. Gallagher, W. Flatley, M. Friggens, A. Evans, R. Loehman, S. Hedwall, L. Brandt, M. Janowiak, and C. Swanston. 2022. Adaptation Strategies and Approaches for Managing Fire in a Changing Climate. Climate 10:58. Schroeder, H.W., and L.M. Anderson. 1984. Perception of Personal Safety in Urban Recreation Sites. Journal of Leisure Research 16:178-194. Schroeder, T.D. 1982. The Relationship of Local Park and Recreation Services to Residential Property Values. Journal of Leisure Research 14, 3:223-234. Schuster, J. L. 2001. Soil and vegetation management: Keys to water conservation on rangeland. Technical Report B-6040, Texas Agricultural Extension Service. Schwantes, A.M., Parolari, A.J., Swenson, J.J., Johnson, D.M., Domec, J.C., Jackson, R.B., Pelak, N. and Porporato, A., 2018. Accounting for landscape heterogeneity improves spatial predictions of tree vulnerability to drought. New Phytologist, 220(1), pp.132-146. Schwantes, A. M., J. J. Swenson, M. González‐Roglich, D. M. Johnson, J. C. Domec, and R. B. Jackson. 2017. Measuring canopy loss and climatic thresholds from an extreme drought along a fivefold precipitation gradient across Texas. Global Change Biology 23:5120- 5135. Schwantes, A.M., Swenson, J.J. and Jackson, R.B., 2016. Quantifying drought-induced tree mortality in the open canopy woodlands of central Texas. Remote Sensing of Environment, 181, pp.54-64. Scott, J. H. (2005). Standard fire behavior fuel models: a comprehensive set for use with Rothermel's surface fire spread model, US Department of Agriculture, Forest Service, Rocky Mountain Research Station. Sedjo, R., and B. Sohngen. 2012. Carbon Sequestration in Forests and Soils. Annual Review of Resource Economics 4:127-144. Siglo Group. 2021. The Green Infrastructure Strengths & Gaps Assessment. City of Austin. Simmons, M. T. 2015. Climates and Microclimates: Challenges for Extensive Green Roof Design in Hot Climates. Pages 63-80 in R. K. Sutton, editor. Green Roof Ecosystems. Springer International Publishing, Cham. 126 Smith, R. 1996. Ecology and Field Biology, 5th edition. Harper Collins College Publishers, New York, NY. Soussana, J. F., P. Loiseau, N. Vuichard, E. Ceschina, J. Balesdent, T. Chevallier, and D. Arrouays. 2004. Carbon cycling and sequestiration opportunities in temperate grasslands. Soil Use and Management:219-230 Stambaugh, M.C., Guyette, R.P., Stroh, E.D., Struckhoff, M.A. and Whittier, J.B., 2018. Future southcentral US wildfire probability due to climate change. Climatic Change, 147, pp.617-631. Stambaugh, M. C., B. O. Knapp, and D. C. Dey. 2021. Fire Ecology and Management of Forest Ecosystems in the Western Central Hardwoods and Prairie-Forest Border. Pages 149-199 in C. H. Greenberg and B. Collins, editors. Fire Ecology and Management: Past, Present, and Future of US Forested Ecosystems. Springer International Publishing, Cham. Stephens, S. L., A. L. Westerling, M. D. Hurteau, M. Z. Peery, C. A. Schultz, and S. Thompson. 2020. Fire and climate change: conserving seasonally dry forests is still possible. Frontiers in Ecology and the Environment 18:354-360. Stovall, A.E., Shugart, H. and Yang, X., 2019. Tree height explains mortality risk during an intense drought. Nature Communications, 10(1), p.4385. Swanston, C. W., M. K. Janowiak, L. A. Brandt, P. R. Butler, S. D. Handler, P. D. Shannon, A. Derby Lewis, K. Hall, R. T. Fahey, L. Scott, A. Kerber, J. W. Miesbauer, and L. Darling. 2016. Forest Adaptation Resources: climate change tools and approaches for land managers. 2nd ed. U.S. Department of Agriculture, Forest Service, Northern Research Station. Talbot, J.F., and R. Kaplan. 1986. Judging the Sizes of Urban Open Areas: Is Bigger Always Better? Landscape Journal 5, 2:83-92. Thurow, T.L., W. Blackburn, S. Warren, and C. Taylor Jr. 1987. Rainfall interception by midgrass, shortgrass, and live oak mottes. Journal of Range Management 40: 455-459 Thurow, T. L. and J. W. Hester. 1997. How an increase or reduction in juniper cover alters rangeland hydrology. Pages 9-22 in 1997 Juniper Symposium. Texas A&M Research and Extension Center, San Angelo, Texas. Tingley, M., V. Ruiz-Gutiérrez, R. Wilkerson, C. Howell, and R. Siegel. 2016. Pyrodiversity promotes avian diversity over the decade following forest fire. Proceedings. Biological sciences 283. TPWD. 2012. Texas Conservation Action Plan 2012 – 2016: Texas Blackland Prairies Handbook. Connally W, editor. Austin, Texas: Texas Parks and Wildlife Department. Tyrväinen, L., and A. Miettinen. 2000. Property Prices and Urban Forest Amenities. Journal of Environmental Economics and Management 39:205-223. Tsunetsugu, Y., B.J. Park, and Y. Miyazaki. 2010. Trends in Research Related to “Shinrin-Yoku” (Taking in the Forest Atmosphere or Forest Bathing) in Japan. Environmental Health and Preventive Medicine 15, 1:27-37. Tsunetsugu, Y., J. Lee, B.-J. Park, L. Tyrväinen, T. Kagawa, and Y. Miyazaki. 2013. Physiological and Psychological Effects of Viewing Urban Forest Landscapes Assessed by Multiple Measurements. Landscape and Urban Planning 113:90-93. Twidwell, D., C. L. Wonkka, C. A. Taylor Jr, C. B. Zou, J. J. Twidwell, and W. E. Rogers. 2014. Drought‐ induced woody plant mortality in an encroached semi‐arid savanna depends on topoedaphic factors and land management. Applied Vegetation Science 17:42-52. Turner, M. G., R. H. Gardner, and R. V. O'Neill. 2001. Landscape Ecology in Theory and Practice. Springer- Verlag, New York, NY. 127 Ulrich, R.S. 1986. Human Responses to Vegetation and Landscapes. Landscape and Urban Planning Ulrich, R.S., and D.L. Addoms. 1981. Psychological and Recreational Benefits of a Residential Park. 13:29-44. Journal of Leisure Research 13, 1:43-65. reduce wildfire risk. Fact Sheet. U.S. Department of Agriculture-Forest Service. 2022. Management to improve forest resilience and van Praag, H., B.R. Christie, T.J. Sejnowski, and F.H. Gage. 1999. Running Enhances Neurogenesis, Learning, and Long-Term Potentiation in Mice. Proceedings of the National Academy of Sciences of the U.S.A. 96, 23: 13427-431. Weaver, J. E. 1954. North American Prairie. Johnsen Publishing, Lincoln, Nebraska. Weaver, J. E. 1968. Prairie plants and their environment. University of Nebraska Press, Lincoln, Nebraska. Whisenant, S. G. 2002. Repairing Damaged Wildlands. A Process-oriented, Landscape Scale Approach. Cambridge University Press, United Kingdom. White, J. D., et al. (2009). The Balcones Canyonlands Preserve Fire Risk and Management: Characterization of woodland fuels and simulated fire behavior in the wildland-urban interface. Report presented to the City of Austin Wildland Conservation Division. Wilcox, B.P. and Haung, Y. 2010. Woody plant encroachment paradox: Rivers rebound as degraded grasslands convert to woodlands. Geophysical Research Letters, vol 37, L07402, doi:10.1029/2009GL041929, 2010 Will, R. E., S. M. Wilson, C. B. Zou, and T. C. Hennessey. 2013. Increased vapor pressure deficit due to higher temperature leads to greater transpiration and faster mortality during drought for tree seedlings common to the forest–grassland ecotone. New Phytologist 200:366-374. Williams, A. P., C. D. Allen, A. K. Macalady, D. Griffin, C. A. Woodhouse, D. M. Meko, T. W. Swetnam, S. A. Rauscher, R. Seager, H. D. Grissino-Mayer, J. S. Dean, E. R. Cook, C. Gangodagamage, M. Cai, and N. G. McDowell. 2013. Temperature as a potent driver of regional forest drought stress and tree mortality. Nature Climate Change 3:292-297. Williams, K.J.H., and J. Cary. 2002. Landscape Preferences, Ecological Quality, and Biodiversity Protection. Environment and Behavior 34, 2: 257-274. Wolch, J.R., J. Byrne, and J.P. Newell. 2014. Urban Green Space, Public Health, and Environmental Justice: The Challenge of Making Cities ‘Just Green Enough’. Landscape and Urban Planning 125: 234-244. Wonkka, C.L., Twidwell, D., West, J.B. and Rogers, W.E., 2016. Shrubland resilience varies across soil types: implications for operationalizing resilience in ecological restoration. Ecological Applications, 26(1), pp.128-145. Xu, X., Polley, H.W., Hofmockel, K. and Wilsey, B.J., 2017. Species composition but not diversity explains recovery from the 2011 drought in Texas grasslands. Ecosphere, 8(3), p.e01704. Yachi, S. and Loreau, M., 1999. Biodiversity and ecosystem productivity in a fluctuating environment: the insurance hypothesis. Proceedings of the National Academy of Sciences, 96(4), pp.1463-1468. Young, D. J. N., J. T. Stevens, J. M. Earles, J. Moore, A. Ellis, A. L. Jirka, and A. M. Latimer. 2017. Long- term climate and competition explain forest mortality patterns under extreme drought. Ecology Letters 20:78-86. 128