20240807-004: WPD EII Quality Assurance Project Plan Part 1 — original pdf
Backup
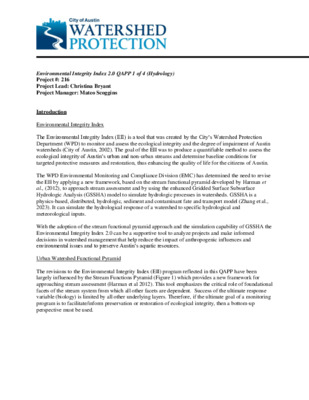
Environmental Integrity Index 2.0 QAPP 1 of 4 (Hydrology) Project #: 216 Project Lead: Christina Bryant Project Manager: Mateo Scoggins Introduction Environmental Integrity Index The Environmental Integrity Index (EII) is a tool that was created by the City’s Watershed Protection Department (WPD) to monitor and assess the ecological integrity and the degree of impairment of Austin watersheds (City of Austin, 2002). The goal of the EII was to produce a quantifiable method to assess the ecological integrity of Austin’s urban and non-urban streams and determine baseline conditions for targeted protective measures and restoration, thus enhancing the quality of life for the citizens of Austin. The WPD Environmental Monitoring and Compliance Division (EMC) has determined the need to revise the EII by applying a new framework, based on the stream functional pyramid developed by Harman et al., (2012), to approach stream assessment and by using the enhanced Gridded Surface Subsurface Hydrologic Analysis (GSSHA) model to simulate hydrologic processes in watersheds. GSSHA is a physics-based, distributed, hydrologic, sediment and contaminant fate and transport model (Zhang et al., 2023). It can simulate the hydrological response of a watershed to specific hydrological and meteorological inputs. With the adoption of the stream functional pyramid approach and the simulation capability of GSSHA the Environmental Integrity Index 2.0 can be a supportive tool to analyze projects and make informed decisions in watershed management that help reduce the impact of anthropogenic influences and environmental issues and to preserve Austin’s aquatic resources. Urban Watershed Functional Pyramid The revisions to the Environmental Integrity Index (EII) program reflected in this QAPP have been largely influenced by the Stream Functions Pyramid (Figure 1) which provides a new framework for approaching stream assessment (Harman et al 2012). This tool emphasizes the critical role of foundational facets of the stream system from which all other facets are dependent. Success of the ultimate response variable (biology) is limited by all other underlying layers. Therefore, if the ultimate goal of a monitoring program is to facilitate/inform preservation or restoration of ecological integrity, then a bottom-up perspective must be used. Figure 1. Stream Functions Pyramid (Harman et al. 2012). A framework for approaching stream assessment. Applied Watershed Research staff have modified the functional pyramid to apply more directly to the urban environment and to watersheds as a unit instead of streams. The most basic difference is that Hydraulics and Geomorphology have been integrated into one layer. Figure 2. EII 2.0 modified watershed functional pyramid. Each layer in this pyramid has a distinct modeling and monitoring approach. This implicitly includes geology as the underlying and fundamental framework for this pyramid, and the climate around it which drives the hydrologic cycle and many other factors that affect all layers. Hydrology Monitoring As the base of the stream functional pyramid, hydrology occupies a central role in the Environmental Integrity Index. It provides the mechanism by which sediment, constituents, and biotic life are transferred throughout the system. Furthermore, knowing how the hydrology of a basin is allocated (water balance, infiltration, runoff ratio, etc.) can be an indicator of its natural state. The EII incorporates hydrologic information into its monitoring program through the combination of infiltration modeling and streamflow modeling calibrated through a set of stream flow gauges. Modeling streamflow time series is a standard tool for assessing the hydrology of a system and can thus be considered as essential in a watershed health monitoring program. The WMS computer software provides streamflow time series predictions at finite points along a stream network for any time period. This makes it ideal for reconstructing past streamflow time series that can then be used to statistically establish thresholds and, thus, to determine whether the hydrologic system is within “control”. Similarly, predictions of the streamflow time series can be evaluated to test correlations with other EII parameters, such as geomorphology, chemistry, and biology. Infiltration modeling is the second method by which watershed hydrology is assessed and monitored. How infiltration is allocated among the amount of runoff and of evapotranspiration can inform users to the health of the watershed. However, it is a difficult parameter to monitor physically. Pedotransfer functions are typically used, but for EII, it will instead be modeled using WMS, since the modeling can capture past amounts of infiltration. The downside to modeling infiltration is that it has no existing method of calibration. WMS is a quasi-3D watershed model that incorporates land use, soil type, and topography into a finite grid to produce flow along the stream through precipitation loading. It also has the ability to simulate groundwater mechanics using physics-based equations. This places this software in the unique position of calculating streamflow and infiltration. Precipitation Monitoring Precipitation represents a major portion of the hydrologic cycle. Historically, arithmetic averages, Thissen polygons or isohyets were employed to distribute point rainfall data across areas between gauges. Accuracy issues often arose from the limited size of gauge networks (Dunne and Leopold 1978). Errors in estimated precipitation across basins were observed to drastically reduce when radar data is calibrated to gauge data (Brandes 1975). Accurate precipitation data is a major control on the accuracy of modeled flow. As such, precipitation monitoring will occur at selected locations as a check or validation of gauge adjusted radar rainfall (GARR) values. Project History The EII is a comprehensive biological, chemical and physical monitoring tool designed to monitor and assess the ecological integrity and the degree of impairment of Austin’s watersheds. It was originally developed and tested in the urban watersheds in 1994 and 1995 and initiated citywide in 1996. By the year 2000, water quality sampling was conducted quarterly, and the biological and habitat surveys were completed annually. Fifty of the City of Austin planning watersheds were grouped into three phases with approximately 150 total sites sampled on a three-year rotating basis (~50 sites sampled per year). Phase 1 primarily included the urban watersheds sampled historically under the Water Watchdog volunteer program (Project 20) while Phase 2 and Phase 3 included suburban and developing watersheds. In 2009, the watersheds were regrouped into two phases for sampling on a two-year rotating schedule. This regrouping was designed to increase site visit frequency to improve the resolution of temporal trend evaluation and align with frequency requirements of the Clean Rivers Program of the Texas Commission on Environmental Quality. Although the methods have remained relatively stable over time, the project has evolved slowly due to advancements in technology, staff bandwidth, and state-of-the-science. A retrospective of the project after 25+ years of work indicated that much could be gained with strategic advancements to the program. The EII has previously been used in the Department’s Master Plan process to evaluate water quality conditions of Austin’s watershed. Additionally, it has been used to satisfy a portion of the City’s National Pollution Discharge Elimination System (NPDES) permit. Perhaps the most valuable use of the EII is as a long-term dataset that can substantiate a baseline condition from which models can be developed. Objective Zero (Herrington 2017), a concept which WPD uses to identify target conditions for aspirational environmental goals, could not be efficiently informed by the structure of EII as it existed in 2022. The 2022 fiscal year was the last year of traditional phase-based quarterly water quality sampling. Field and analysis methods were developed through four subcommittees: Hydrology, Geomorphology, Physicochemical and Biology. Each subcommittee evaluated the EII program for the past 30 years, reviewed the relevant body of work (both COA projects and literature), and discussed goals and aspirations to meet the needs (both current and future) of the rapidly growing community in the context of climate change and evolving environmental stressors. Previous EII Methodology and Related Reports • Environmental Integrity Index Methodology CM-99-01. • Flow and impervious cover thresholds in aquatic communities in Austin SR-15-12. • Growth Trends and Environmental Integrity in Austin, Texas (2011) CN-11-02 • Creation of a multi-metric index for describing the environmental integrity of Austin-area lakes • Water Resource Evaluation Standard Operating Procedures Manual SR-04-04, revised 2015. (2011) SR-11-19. Project Objectives The objectives of this QAPP are: 1. Provide additional calibration data for citywide hydrology models at 7 new EII 2.0 sites. 2. Validate current calibrated citywide hydrology modeling for the 2 existing USGS sites. 3. Verify precipitation data at 7 targeted sites. (Two EII 2.0 sites are managed by the USGS and will not have precipitation gauges.) 4. Collect continuous stage data to calculate discharge at 9 targeted sites for use with other components of EII 2.0 (Hydraulics/ Geomorphology, Physicochemical). Policy Relation Results from the EII 2.0 monitoring program will be used as an environmental input to the Watershed Health Decision Support System (WHDSS). This system is a part of the Department’s Strategic Plan in prioritizing its work in either improving or preserving stream conditions throughout the City, while incorporating equity. Specifically, through WHDSS, the EII will identify those streams that require environmental mitigation or protection (or some combination of both). Using the Hydrology QAPP, streams will be monitored for flow and stage at various locations to support hydrologic modeling. The output of the modeling will be hydrographs and infiltration estimates throughout the city. This output can be reported and scored for problems, such as flashiness or low baseflow. Additionally, the outputs can also be used to support further modeling of sediment transport and water quality, as well as used in statistical modeling of Benthic Macroinvertebrate and Diatom metrics. In this way, the Hydrology QAPP is the keystone of the EII 2.0 monitoring program and in informing the environmental outcomes for Austin’s creeks. Project Committee The EII 2.0 project has four separate QAPP committees structured in accordance with the modified functional pyramid: Hydrology, Hydraulics/ Geomorphology, Physicochemical, and Biology. The hydrology committee structure (ARCI) is detailed in Table 1. Table 1. EII 2.0 Hydrology QAPP Committee Role Personnel Planning Data Collection I Analysis Modeling Report Writing A I I Project Manager Project Lead Data Manager Committee Member (DA/DS) Committee Member (DA/DS) Committee Member (DA/DS) Committee Member (DA/DS) Committee Member (DA/DS) Committee Member (AWR) Committee Member (AWR) Committee Member (Other subcommittees) Mateo Scoggins Christina Bryant Rob Clayton Young-Hoon Jin Abel Porras Yazmin Avila Andrew Chu Harshita Mahaseth C Ryan Burke Staryn Wagner Andrew Clamann, Angel Santiago, Zhen Xu A R C R R C C R C C A R C C I I I R C C A I R R R R R C I C A I R R R R R I I C R I C R I I I C I C * A = Accountable (ensures project has needed resources, held accountable if not completed); R = Responsible (leads development of study components, performs and delegates tasks, reviews deliverables before deemed complete); C = Consulted (specialist in specific component of study, available for comments); I = Informed (kept in the loop, comments optional) Personnel Time Table 2. Estimated Time Budget Task Project Management Hydrology Modeling Personnel Christina Bryant DA/DS Staff Work Time Estimated 80hrs annually Estimated 500hrs annually Site Design and Construction* AWR Staff Estimated 500hrs Field Equipment Maintenance* AWR Staff Estimated 500hrs annually Hydrology Data Management AWR Staff Estimated 250hrs annually Data Management Reporting DA/DS (various) AWR Staff Estimated 200hrs Estimated 40hrs annually * Task hours to be shared with Physio-Chemical QAPP Project Funding This project is 100% funded by the AWR operating budget. Table 3. Project Funding Sources FDU (Fund Department Unit) 5100 6300 3140 Project Schedule Table 4. Project Schedule Target FY24-Q1 FY24-Q2 FY24-Q3 FY24-Q4 Hydrology Data Collection Field data QA/QC Hydrograph QA/QC Modeling and data analysis Annual report drafting Annual report review Routine discharge measurements for rating curve validation and site maintenance and calibration checks of precipitation equipment ✓ ✓ ✓ ✓ ✓ ✓ ✓ ✓ ✓ FY25- Q1 Oct- 24 FY25- Q1 Nov- 24 FY25- Q1 Dec- 24 ✓ ✓ ✓ ✓ ✓ Field and Experimental Methods Site Selection Candidate monitoring sites were selected by hierarchical cluster analysis, which seeks to partition all identified nodes in Austin watersheds into distinct groups with similar observations. The cluster analysis used the following watershed characteristics: • Soil Survey Geographic Database (SSURGO) soil infiltration data • Annual total nitrogen and phosphorus loading • Erosive potential by areal slope • Flashiness of the storm events by percent impervious cover Cluster analysis identified eight candidate sites (Table 5). The project team completed field evaluations at the sites to determine suitability for access and installation of monitoring station. One additional site was added to monitor the effects from discharges from wastewater treatment facilities to the receiving water. Table 5. Continuous Monitoring Site Locations Watershed Site ID Site Short Name 51 Barton @ Lost Creek Blvd 142 Bull Creek @ Spicewood Springs Rd 7th Crossing 2956 Onion @ Ped Xing south of William Cannon 3068 Elm @ FM969 1192 Gilleland @ FM 973 233 Rinard @ Bradshaw Rd 623 Slaughter @ FM1826 5285 Walnut @ Metric Blvd BAR BUL ELM GIL ONI RIN SLA WLN WLS Cluster ID 2 5* 4 5 8 1 3 6 7 463 Wells Branch @ Walnut Creek Park Rd * Although site 1192 is located within one of the eight clusters of the model, this site has been selected to characterize stream segments that are wastewater effluent driven. Figure 3. Locations of EII 2.0 monitoring sites The preference is to input the new EII 2.0 sites to existing sites in the WRM database, so new data can be compared to historic data. All EII 2.0 sites will be inputted as the nearest existing EII 1.0 site unless a site does not exist at that location or exceeds a reasonable distance (~50 feet) to be listed as the same site. If this occurs a new site will be added to the database. Note that since site equipment must be mounted near solid structures, in some instances this tolerance was exceeded. Site Equipment Location Each of the 9 sites will be equipped with instrumentation for continuous stage monitoring, peak flow verification, continuous water quality monitoring, and automated discrete water quality sampling. Equipment will be placed in the best location considering the following constraints: - The shelter must be located outside of the floodplain to prevent floodwater contacting the wiring and battery. - The maximum tubing length the autosampler pump can overcome is 100 feet. - The maximum head the autosampler pump can overcome is 28 feet. The location of the equipment shelter, as influenced by the above constraints, will also influence the location of the stage sensor (bubbler and orifice or ultrasonic sensor); however, the stage sensor location is otherwise independent of the water quality equipment in channel. The stage sensor will be installed in a location that minimizes the likelihood of equipment loss during flow events, prevents jumps in the hydrograph, minimizes pileup and drawdown effects from structures within the channel, and receives equivalent flow as at the location of the water quality equipment (i.e., no inputs or outputs to flow will occur between these two locations). The autosampler intake line will be installed at the channel low point, as determined by a survey of the channel. The Sonde will be affixed to a nearby semi-protected, well-mixed location. Site Equipment This equipment represents that used for hydrologic monitoring only. For a list of water quality equipment see the EII 2.0 Physio-Chemical QAPP. ISCO Signature Flow meter ISCO Tipping Bucket Rain gauge • • • Remote Bubbler Module • Crest stage gauge (CSG) • Ultrasonic Sensor • Laserflow Flowmeter Data Storage and Quality Assurance The ISCO Signature meter located at each site is equipped with cellular modems that push data on a scheduled 6-hour interval for baseflow, and 15 minutes when storm flows are detected utilizing a Flowlink IP Listener configured on the COA server WPD_FIELDMONTR. Data from this server is uploaded to HYDSTRA servers on a 24-hour basis via a scheduled script. This generates a log file stored on the T:\ drive, which is regularly reviewed by the DA/DS team for equipment malfunction and communication issues. The unedited data is then moved into an “M” file on the Data Managers Workbench where AWR staff perform quality checks. Quarterly, AWR staff will perform the screening process detailed in the StormSOP. This process looks for gaps (201, 151) or unreliable data based on best judgment and known site hydraulics. To remediate error codes or questionable data, the following codes are used to flag for consideration when being used in calculations or modeling. Figure 4. Data codes used during the quarterly review process, as per the Storm SOP. Once the quarterly review process is complete and data corrections have been applied the approved data is archived and used in analysis. Hydrology Experimental Methods The experimental method for hydrology described herein exists only for the purpose of calibrating and validating the watershed models. The method consists of accessing the continuous time series data generated by USGS and City of Austin stream gauges in Austin creeks, calculating the daily average, and adjusting model parameters to fit the daily average. Four metrics were used to evaluate the fit of the model to the observed data (see the Calibration and Verification of Modeled Hydrology section below). Modeling and Analytical Methods The Watershed Modeling System (WMS) interface with the physically based, distributed-parameter hydrologic model, Gridded Surface Subsurface Hydrologic Analysis (GSSHA), will be used to estimate the hydrology at 3000’ intervals. The results are based on overland flow equations, in-channel flow equations, and unsaturated sub-surface flow equations. Inputs to the model include 15-min precipitation and 1-hour meteorological data on an annual basis, the existing land use, soil types, impervious cover, and elevations of the terrain. The outputs include a hydrograph at each of the 3000’ points as well as an arial map of simulated cumulative infiltration. Calibration and Verification of Modeled Hydrology Table 6. Parameters to be calibrated for hydrology Parameter Reference Process Overland flow Roughness Stream flow Roughness Infiltration (GAR: Green & Ampt with Redistribution) Evapo- transpiration Groundwater Saturated Hydraulic Conductivity, Capillary Head, Effective Porosity, Pore Index, Residual Saturation, Field Capacity Saturation, Wilting Point Saturation, Initial Soil Moisture Land surface albedo, Vegetation height, Vegetation transmission coefficient, Canopy stomatal resistance Hydraulic Conductivity, Porosity 5.2.2 Overland Flow Hydraulic Roughness: https://gsshawiki.com/Surface_Water_Routing:O verland_Flow_Routing Chow, V.T. 1959. Open-channel hydraulics. New York: McGraw Hill Infiltration: Parameter Estimates: https://gsshawiki.com/Infiltration:Parameter_Esti mates Gridded Surface Subsurface Hydrologic Analysis (GSSHA) User’s Manual, Pages 120 - 126 Geologic and Hydrogeologic Framework of Regional Aquifers (1996) Metrics for Modeled Hydrograph Calibration/Verification (Moriasi et al. 2007), for a monthly time step: - R2 (coefficient of determination) > 0.8 - RSR (RMSE-observations Standard deviation Ratio) ≤ 0.5 - NSE (Nash-Sutcliffe Efficiency) > 0.75 - PBIAS (Percent BIAS) < ±10 Site Discharge Calculations Discharge (q, ft3/s) at each site will be calculated from Manning’s equation, which determines velocity in open channel flow from physical site characteristics: where v is velocity (ft/s), n is Manning’s roughness coefficient, R is the hydraulic radius of the channel (ft), and S is the channel bed slope. R is calculated from: 𝑣 = 1.49 𝑛 2 3𝑆 1 2 𝑅 𝑅 = 𝑎 𝑃 where a is the channel cross-sectional area (ft2) and P is the wetted perimeter (ft). Once velocity is determined, q can be calculated as: q = v × a All discharge equations and site-specific values for n (Manning’s roughness coefficient), R (hydraulic radius), and S (channel slope), will be housed in HYDSTRA. Rating curve development from calculated discharge is described below. Table 7. Site Discharge Equations Stage Equipment Type and Location(s) Discharge Equation(s) ID RIN BUL ELM WLS SLA BAR WLN ONI GIL Ultrasonic sensor: Downstream side of bridge, mounted over creek centerline Bubbler orifice: Upstream of box culvert, affixed to surveyed low point of channel bottom Ultrasonic sensor: Downstream side of bridge, mounted over creek centerline Bubbler orifice: Upstream side of dam (located 100 ft downstream of bridge), affixed to surveyed low point in center of dam outflow Site managed by USGS Site managed by USGS Ultrasonic sensor: River right, mounted to pedestrian bridge over gauge pool Bubbler orifices: 1) Upstream side of box culvert, affixed to surveyed low point of first culvert opening from river left; 2) Upstream side of box culvert, affixed to structure river right of orifice 1 Bubbler orifice: Downstream side of bridge pile, adjacent to LCRA equipment Open channel flow using Manning’s equation 1) Box culvert flow using Manning’s equation; 2) Open channel flow using Manning’s equation (when bridge overtops) Open channel flow using Manning’s equation Open channel flow using Manning’s equation (flow only occurs over the dam) N/A, rating curve managed by USGS N/A, rating curve managed by USGS 1) Open channel flow using Manning’s equation; 2) Open channel flow using Manning’s equation (when pedestrian bridge overtops) 1) Culvert flow using Manning’s equation; 2) Open channel flow using Manning’s equation (when all culverts fill) N/A, rating curve developed by LCRA, to be verified by site discharge measurements Site Level and Discharge Data Quality Objectives and Quality Assurance Assuming the channel is stable, periodic site visits will occur at a maximum every 8 weeks, to check site equipment and confirm that the channel has not undergone changes that invalidate the stage-discharge relationship. More frequent site visits (up to weekly) may occur while the stage discharge relationship is being constructed or if the channel is deemed unstable (Wahl et al. 1995). Discharge measurements will be used to validate rating curves at each site. Measurements will be collected during a variety of flow regimes; however, EII 2.0 is concerned with baseflow, and as such, validation will be weighted towards the low and mid flow portions of the rating curve. Total discharge for the cross section will be computed using the velocity-area midsection method. Depending on the depth at each specific vertical subsection, the two-point method or the 0.6-depth method will be used to measure a representative velocity at that subsection along the channel cross section (Turnipseed and Sauer 2010). Depending on site conditions, alternative discharge measurement methods can be employed, such as the use of portable flumes, portable weirs, or the volumetric method (Turnipseed and Sauer 2010; Seybold et al. 2023). If user error is suspected or equipment error occurred during the discharge measurement, a check measurement will be collected at a different cross section using different equipment, as appropriate and available (Turnipseed and Sauer 2010). Up to three measurements will be collected; the first of any two measurements to agree will be reported. However, if three measurements are collected and two do not agree a verification resample will be performed at a different time (see the StormSOP for more information). Reported discharge measurements will be compared to the confidence band around each site’s rating curve (see Stage-Discharge Rating Curve Development section below). To prevent measurement bias field staff will have no knowledge of the discharge confidence interval at the time of the site visit. Discharge measurements falling outside the confidence band will suggest either changes in the channel, equipment issues, or that a random error has occurred. In the event of a confidence band exceedance an independent verification resample will be collected when the flow is changed, generally at least one week later. If the verification resample exceeds the confidence band, then actions to develop a new rating curve may be taken. If the verification resample does not exceed the confidence band, then there was a random error, and no action is required. In this vein, a check measurement performs quality control on the individual measurement, while a verification resample validates statistical assumptions concerning a lack of autocorrelation between measurements (USEPA 2009). Field safety best practices will be followed (Yobbi et al. 1996). As such, wading discharge measurements will not be collected if deep and/ or swift water are observed at the site during inspections. USGS recommends avoiding wading measurements when the product of depth (ft) and water velocity (ft/ sec) exceeds 8 at any location in the channel cross section (Meals and Dressing 2008); City of Austin personnel will adhere to this rule as well. A crest stage gage (CSG) will be affixed at a targeted location within the 25-year floodplain at each site to record peak stage when it is unsafe for field personnel to measure discharge (Sauer and Turnipseed 2010). Standard USGS practices for CSG operation and maintenance will be followed (Friday 1965). Staff plates will be affixed at sites where rating curve development is expected to be complex (e.g., split flow through multiple culverts, multiple rating curves required for culvert and open channel flow at the same site, sites with shifting beds, sites with the potential for rapid rises in stage, etc.). The following sites will have staff plates installed: ELM (Elm @ FM 969), WLN (Walnut @ Metric Blvd), ONI (Onion @ Ped Xing), GIL (Gilleland @ FM 973). Staff plates will be used to manually check data from the continuous stage sensor and keep field personnel aware of changing conditions during discharge measurements. Staff plate readings will be recorded during the discharge site visit. Accurate stage data is dependent on an accurate and reliable datum. Each site will have an arbitrary, site- specific datum established, based on three stable, independent reference marks, which are also independent of any gauge infrastructure or control feature. A preference will be given towards rock outcrops and masonry structures, if possible, at each site. If such locations are not available, rebar posts will be driven at least 24” into the ground and affixed with concrete to establish a stable reference point. Rebar driven into clay soils will be avoided, as shrink-swell processes in clays do not provide a stable datum. Differential levels (Kenney 2010) will be run on a yearly basis to check that the bubbler orifice, ultrasonic senor, CSG, and staff plate(s) (if applicable) are stable within the datum. The location and description of all index points on the gauges (e.g., a specific bolt head on the staff plate, etc.) and reference points will be stored in HYDSTRA. USGS recommends running levels on a yearly basis until a stable datum is established, and then moving to a 3-year timeframe; given the short nature of this project, preference towards accurate real-time data over corrected historic data, and abundance of clay-rich soils across east Austin, levels will remain on a one-year timeframe. Circuit closure errors will follow USGS standards and will be distributed to the surveyed locations to yield adjusted elevations (Kenney 2010). If site equipment is replaced or a new rating curve is developed, a differential level survey will also be run to tie the new datum to the previous one. If the change in adjusted elevation of the bubbler orifice, ultrasonic sensor, CSG index point, and/ or staff plate at any site is more than +/- 0.02’ between differential levels surveys, a datum review process will be initiated at that site. Although USGS tolerance is +/- 0.015’ for gauge adjustments, +/- 0.02’ will be used throughout this project, as the assumed accuracy limits of the model do not warrant such a low threshold. During this review process AWR staff will determine if the stage equipment moved enough to change calculated discharge from observed discharge at any point on the rating curve by more than +/- 0.5 cfs (the assumed accuracy of the model). If this +/- 0.5 cfs threshold is exceeded, the rainfall runoff curve and hydrograph will be inspected to determine the timing of equipment movement (e.g., potential major event(s), such as a flood). If such an event can be identified, a constant correction in the amount of the surveyed movement will be applied to historic stage data from the time of the event to the time of the differential levels survey. If an event cannot be identified, a prorated correction will be applied between levels surveys. After the correction is applied to stage data, discharge will be recalculated, and the model will be rerun using the updated discharge values. All future stage data will have a constant correction applied forward, unless an additional +/- 0.02’ movement within the datum is observed, at which point the review process will be reinitiated. Note that datum corrections will only be applied to stage equipment with documented movement, and not uniformly to all stage equipment at a site. I.e., if the CSG but not the bubbler orifice is determined to have moved at a site, a correction will only be applied to stage data collected from the CSG, and not that collected from the bubbler. Stage-Discharge Rating Curve Development Using the channel cross-sectional survey and an estimate of roughness, flow will be calculated at each stage in the channel using Manning’s Equation. A rating curve for each site will then be developed using non-linear regression of level to calculated flow. The nonlinear regression format will use the following curve: 𝑄 = 𝑎(ℎ − 𝐺𝑍𝐹)𝑏 where Q is discharge (ft3/s), h is stage (ft), GZF is gauge of zero flow (ft), and a and b are constants to be estimated at each site to mimic the flow-level relationship provided by Manning’s Equation. The a and b coefficients will be based on minimizing errors between the curve and Manning’s Equation. This process will be repeated for high and low estimates of roughness. Based on this range of roughness estimates, an upper and lower confidence band will be determined for each site. Over time, actual field measurements of flow and level will be observed and compared to the confidence band as a method of calibrating the curve. From this, the accuracy of the confidence bands can be assessed from variations due to seasons. Manning’s coefficients will be selected to generate discharges with the least errors to the rating curves, reflect conditions of vegetation and seasons, and enclose as much observed data as possible within the confidence intervals. Precipitation data quality objectives and quality assurance Precipitation data for model input will be obtained from Gauge-Adjusted Radar Rainfall (GARR), which provides a gridded dataset at 1 km resolution for radar rainfall that is adjusted to values recorded at precipitation gauges. The Austin-area grid uses gauge data from networks managed by the City of Austin FEWS group, the Lower Colorado River Authority (LCRA), the Upper Brushy Creek Water Control and Improvement District, the U.S. Geological Survey (USGS), and the National Weather Survey (NWS). Equipment types and maintenance and calibration schedules vary at locations across the grid. Because of this, seven EII 2.0 sites will have tipping bucket precipitation gauges installed, as a verification of GARR values used in the model. The two USGS sites will not have rain gauges installed. Rainfall verification will be solely based on rainfall-runoff analysis at those sites. EII 2.0 precipitation gauge maintenance activities will include instrument leveling and funnel cleaning (as needed), wet and dry test tips, and removal of overhead obstructions (as feasible), and will occur at least every 8 weeks. Data will be reviewed after each storm to ensure the bucket is not clogged; any suspected clogging will initiate an immediate site visit. Calibration checks will occur on a quarterly basis. If recorded tips differ from the expected value by more than +/- 10% the gauge will be replaced. Rainfall-runoff analysis will be performed for storm events at all sites as an additional verification of the reported GARR data. During this analysis, gridded rainfall data at each station will be compared to the corresponding continuous discharge to ensure that rainfall and runoff are commensurate. A visual screening of the rainfall runoff analysis will occur quarterly; any precipitation data that does not result in an observed discharge will be flagged and not used. Assumptions Hydrology The model calibration includes adjustment of initial parameter values based on references for the land use and soil types. Similarly, some values for the subsurface layers, such as layer depths and hydraulic conductivity of the streambed, will be assumed and calibrated without initial referenced values. Table 8. Model Assumptions Process Card Comments CHANNEL_INPUT Uses assumed trapezoidal cross-sections Stream Flow Infiltration Groundwater M_RIVER K_RIVER SOIL_MOIST_DEPTH TOP_LAYER_DEPTH WATER_TABLE AQUIFER_BOTTOM GW_LEAKAGE_RATE Uniform value (m) for stream sediment thickness for stream losses Uniform hydraulic conductivity (cm/hr) of streambed for stream losses Full depth for soil moisture computations Upper layer depth (included in SOIL_MOIST_DEPTH) for just an upper layer soil moisture layer Specifies the initial water table elevation Specifies the lower extent of the aquifer Leakage rate (cm/hr) through the bottom of the aquifer; a uniform value that is applied to every cell in the grid Assumptions are applied to the entire watershed and values are consistent throughout each watershed. Groundwater is the one exception; the assumed valued will be changed if model recalibration is required. Reporting An annual report will be prepared to summarize progress in data collection and whether model needs are met. A decision will be made then on whether sampling strategies need to be adjusted for the following sample year. Information will be available to the public via a web-based interactive map (www.atxwatersheds.com/findyourwatershed/ or similar) will provide customers with the most current modelled results. Raw data will be reposited in the WRM and Hydstra databases and made available through Socrata portal. Distribution The most recent QAPP is available in the WPD SharePoint. The project lead is responsible for informing all committee members via email if significant changes are made to the QAPP. Change Procedures The Project Lead will maintain this QAPP. Proper document management procedures are followed during any update and the QAPP will be locked for editing. The original approved QAPP will be kept as documentation and any changes to the QAPP are summarized in the QAPP Revision History below. Table 9. QAPP Acceptance Subcommittee Role Name Date of Acceptance Project Manager Mateo Scoggins 11/14/2023 (!) Hydrology Project Lead (QAPP 1 of 4) Christina Bryant 11/09/2023 DA/DS Supervisor Data Manager Abel Porras Rob Clayton 11/16/2023 11/09/2023 Committee Member (DA/DS) Young-Hoon Jin 11/13/2023 Committee Member (AWR) Hydraulics/Geomorphology Project Lead (QAPP 2 of 4) Physicochemical Project Lead (QAPP 3 of 4) Biology Project Lead (QAPP 4 of 4) Ryan Burke Zhen Xu 11/14/2023 11/13/2023 Angel Santiago 11/14/2023 Andrew Clamann 11/13/2023 Table 10. QAPP Revision History Date Modified By Modification Description Date of QAPP Acceptance Subcommittee approval References Brandes EA. 1975. Optimizing Rainfall Estimates with the Aid of Radar. Journal of Applied Meteorology. 14(7):1339–1345. City of Austin, 2002. Environmental Integrity Index Methodology. Environmental Resources Management Division, Watershed Protection Department, City of Austin. Water Quality Report Series COA-ERM 1999-01. https://services.austintexas.gov/watershed_protection/publications/document.cfm?id=186267. Dunne T, Leopold LB. 1978. Water in Environmental Planning. New York: W.H. Freeman and Company. Friday J. 1965. The Operation and Maintenance of a Crest-Stage Gaging Station. [accessed 2023 Jul 8]. https://www.mendeley.com/reference-manager/reader-v2/a38864e1-cc71-3817-90f6- 673302737b4b/34bcbfd9-3c5e-f49d-4658-2e624d85abd4. Harman W, Starr R, Carter M, Tweedy K, Clemmons M, Suggs K, Miller C. 2012. A Function-Based Framework for Stream Assessments and Restoration Projects. Washington, DC Report No.: EPA 843-K- 12-006. Herrington, C.S. 2017. Objective Zero: Improving the water quality mission objectives of the Watershed Protection Department. SR-18-02, City of Austin, Watershed Protection Department. https://services.austintexas.gov/watershed_protection/publications/document.cfm?id=292410 Kenney TA. 2010. Levels at Gaging Stations: U.S. Geological Survey Techniques and Methods Chapter 19 of Book 3, Section A. [accessed 2023 Jul 8]. https://pubs.usgs.gov/tm/tm3A19/tm3A19.pdf. Meals DW, Dressing SA. 2008. National Nonpoint Source Monitoring Program Tech Notes 3: Surface Water Flow Measurement for Water Quality Monitoring Projects. Prentice Hall. [accessed 2023 Jul 8]. https://www.epa.gov/sites/default/files/2016-05/documents/tech_notes_3_dec2013_surface_flow.pdf. Moriasi D, Arnold J, Liew M Van. 2007. Model evaluation guidelines for systematic quantification of accuracy in watershed simulations. Transactions of the. [accessed 2016 Oct 18]. http://elibrary.asabe.org/abstract.asp?aid=23153. Sauer VB, Turnipseed P. 2010. Stage Measurement at Gaging Stations: U.S. Geological Survey Techniques and Methods Chapter 7 of Book 3, Section A. [accessed 2023 Jul 8]. https://pubs.usgs.gov/tm/tm3-a7/tm3a7.pdf. Seybold EC, Bergstrom A, Jones CN, Burgin AJ, Zipper S, Godsey SE, Dodds WK, Zimmer MA, Shanafield M, Datry T, et al. 2023. How low can you go? Widespread challenges in measuring low stream discharge and a path forward. Limnol Oceanogr Lett. doi:10.1002/lol2.10356. [accessed 2023 Oct 15]. https://aslopubs.onlinelibrary.wiley.com/doi/epdf/10.1002/lol2.10356. Turnipseed P, Sauer VB. 2010. Discharge Measurements at Gaging Stations: U.S. Geological Survey Techniques and Methods Chapter 8 of Book 3, Section A. [accessed 2023 Dec 8]. https://pubs.usgs.gov/tm/tm3-a8/tm3a8.pdf. USEPA. 2009. STATISTICAL ANALYSIS OF GROUNDWATER MONITORING DATA AT RCRA FACILITIES UNIFIED GUIDANCE- EPA 530/R-09-007. [accessed 2023 Oct 15]. https://archive.epa.gov/epawaste/hazard/web/pdf/unified-guid.pdf. Wahl KL, Thomas WOJr, Hirsch RM. 1995. Stream-Gaging Program of the U.S. Geological Survey. Reston. [accessed 2023 Oct 15]. https://pubs.usgs.gov/circ/circ1123/index.html. Yobbi DK, Yorke TH, Mycyk RT. 1996. A Guide to Safe Field Operations: U.S. Geological Survey Open-File Report 95-777. [accessed 2023 Jul 8]. https://pubs.usgs.gov/of/1995/of95-777/ofr95777.pdf. Zhang, H., Cao, Q., Ma, Y. 2023. Comparison of two hydro-sediment models based on energy principle of water movement in saturated/unsaturated soils. Energy Reports 9, 1561-1570. [accessed 2023 Oct 17]. https://doi.org/10.1016/j.egyr.2022.11.138. Appendix A Change Log