20240717-002: Hydrilla Management Impacts on a Largemouth Bass Fishery Article for reference — original pdf
Backup
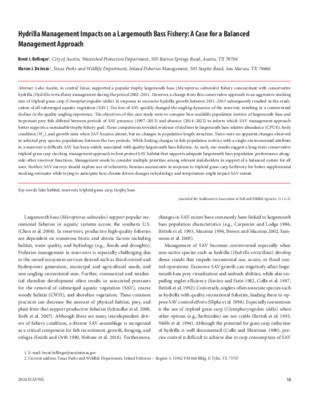
Hydrilla Management and Bass Populations Bellinger and De Jesús Hydrilla Management Impacts on a Largemouth Bass Fishery: A Case for a Balanced Management Approach Brent J. Bellinger 1, City of Austin, Watershed Protection Department, 505 Barton Springs Road, Austin, TX 78704 Marcos J. De Jesús 2, Texas Parks and Wildlife Department, Inland Fisheries Management, 505 Staples Road, San Marcos, TX 78666 Abstract: Lake Austin, in central Texas, supported a popular trophy largemouth bass (Micropterus salmoides) fishery concomitant with conservative hydrilla (Hydrilla verticillata) management during the period 2002–2011. However, a change from this conservative approach to an aggressive stocking rate of triploid grass carp (Ctenopharyngodon idella) in response to excessive hydrilla growth between 2011–2013 subsequently resulted in the eradi- cation of all submerged aquatic vegetation (SAV). The loss of SAV quickly changed the angling dynamics of the reservoir, resulting in a controversial decline in the quality angling experience. The objectives of this case study were to compare how available population metrics of largemouth bass and important prey fish differed between periods of SAV presence (1997–2013) and absence (2014–2022) to inform which SAV management approach better supports a sustainable trophy fishery goal. These comparisons revealed evidence of declines in largemouth bass relative abundance (CPUE), body condition (Wr ), and growth rates when SAV became absent, but no changes in population length structure. There were no apparent changes observed in selected prey species populations between the two periods. While linking changes in fish population metrics with a single environmental attribute in a reservoir is difficult, SAV has been widely associated with quality largemouth bass fisheries. As such, our results suggest a long-term conservative triploid grass carp stocking management approach to best protect SAV habitat that supports adequate largemouth bass population performance along- side other reservoir functions. Management needs to consider multiple priorities among relevant stakeholders in support of a balanced system for all uses. Further, SAV surveys should explore use of volumetric biomass assessments in response to triploid grass carp herbivory for better supplemental stocking estimates while trying to anticipate how climate driven changes in hydrology and temperature might impact SAV extent. Key words: lake habitat, reservoir, triploid grass carp, trophy bass Journal of the Southeastern Association of Fish and Wildlife Agencies 11:14–22 Largemouth bass (Micropterus salmoides) support popular rec- reational fisheries in aquatic systems across the southern U.S. (Chen et al. 2004). In reservoirs, productive high-quality fisheries are dependent on numerous biotic and abiotic factors including habitat, water quality, and hydrology (e.g., floods and droughts). Fisheries management in reservoirs is especially challenging due to the varied ecosystem services desired such as flood control and hydropower generation, municipal and agricultural needs, and non-angling recreational uses. Further, commercial and residen- tial shoreline development often results in associated pressures for the removal of submerged aquatic vegetation (SAV), coarse woody habitat (CWH), and shoreline vegetation. These common practices can decrease the amount of physical habitat, prey, and plant litter that support productive fisheries (Schindler et al. 2000, Roth et al. 2007). Although there are many interdependent driv- ers of fishery condition, a diverse SAV assemblage is recognized as a critical component for fish recruitment, growth, foraging, and refugia (Smith and Orth 1990, Nohner et al. 2018). Furthermore, changes in SAV extent have commonly been linked to largemouth bass population characteristics (e.g., Carpenter and Lodge 1986, Bettoli et al. 1993, Maceina 1996, Brown and Maceina 2002, Sam- mons et al. 2005). Management of SAV becomes controversial especially when non-native species such as hydrilla (Hydrilla verticillata) develop dense stands that impede recreational use, access, or flood con- trol operations. Excessive SAV growth can negatively affect large- mouth bass prey visualization and ambush abilities, while also im- peding angler efficiency (Savino and Stein 1982, Colle et al. 1987, Bettoli et al. 1992). Conversely, anglers often associate species such as hydrilla with quality recreational fisheries, leading them to op- pose SAV control efforts (Slipke et al. 1998). Especially contentious is the use of triploid grass carp (Ctenopharyngodon idella) when other options (e.g., herbicides) are not viable (Bettoli et al. 1993, Webb et al. 1994). Although the potential for grass carp reduction of hydrilla is well documented (Colle and Shireman 1980), pre- cise control is difficult to achieve due to carp consumption of SAV 1. E-mail: brent.bellinger@austintexas.gov 2. Current address: Texas Parks and Wildlife Department, Inland Fisheries – Region 3, 11942 FM 848 Bldg. F, Tyler, TX 75707 14 2024 JSAFWAdecreasing over time, requiring restocking, as well as escapement during floods, difficulty of removal, and potential for SAV over- grazing (Pípalová 2006). Use of grass carp has resulted in complete loss of SAV and corresponding reduction in overall fishery quality (Bettoli et al. 1993, Brown and Maceina 2002, Chilton et al. 2008). Lake Austin in central Texas, USA, is well known for produc- ing trophy-sized (>3.6 kg) largemouth bass (De Jesús and Faroo- qi 2017). During the timeframe encompassed by our study, Lake Austin’s status as a trophy bass fishery was demonstrated by re- corded fishing tournament results and entries into Texas Parks and Wildlife Department (TPWD) angler programs (e.g., Angler Rec- ognition Program and Toyota ShareLunker Program). Eventually, Lake Austin became nationally renowned for its exceptional bass fishery and made headlines amongst the top bass conservation so- cieties in their national top rankings during this period. Prior to 2009, the SAV at Lake Austin was managed by targeting hydrilla coverage through a conservative incremental grass carp stocking strategy, while relying on other beneficial aquatic mac- rophytes to supplement habitat for the bass fishery (Chilton and Magnelia 2008). However, beginning in 2009, there was a regional drought-of-record that reduced cooler flows from hypolimnet- ic releases into Lake Austin, stimulating rapid spread of hydrilla (Shivers et al. 2018), and resulting in increased pressure from dam operators and public stakeholders to deviate from the conserva- tive stocking approach of triploid grass carp. After complying with these demands there was a near complete elimination of SAV after 2013 that has persisted through the present, despite management efforts to restore SAV (Bellinger and Schad 2019). Following the loss of SAV, anglers believed that the largemouth bass fishery in Lake Austin declined and blamed the management authorities for this change. To this end, our goal was to learn from this case ex- perience by analyzing available fisheries data and to develop an improved balanced SAV management approach that would restore a quality trophy fishery at Lake Austin, while considering other user interests. Study Area Lake Austin is a 33 km long, 648-ha mainstem impoundment on the Colorado River in Austin, Texas that was constructed in 1939 (see Chilton and Magnelia 2008). Water levels in Lake Aus- tin are maintained at approximately 150 m above mean sea level but have been periodically drawn down (~3 m) for a few weeks during the winter for SAV control and to allow for maintenance of bulkheads and docks (De Jesús and Farooqi 2017). The reser- voir has an average depth of 3.5 m and a maximum depth of 20 m near the Tom Miller Dam. The reservoir shoreline and riparian habitat are steadily being converted from earthen banks with Hydrilla Management and Bass Populations Bellinger and De Jesús 15 abundant terrestrial and emergent aquatic vegetation (e.g., Typha spp., Schoenoplectus spp., and Justicia americana), and SAV (e.g., Potamogeton spp., Chara spp., Vallisneria americana, Ceratophyl- lum demersum) to hardened revetment, residential lawns, and vegetation-free littoral zones; at the time of this study over 50% of the lakeshore had been developed and hardened (De Jesús and Farooqi 2017). Prior to and during establishment by hydrilla, an- other non-native aquatic plant, Eurasian watermilfoil (Myriophyl- lum spicatum), had been a predominant species and was managed with winter drawdowns. Eurasian watermilfoil competed strongly alongside hydrilla, notably in the upper sections of the reservoir, where inflows from hypolimnetic releases from Lake Travis main- tain cooler water temperature (Smith and Barko 1990). Hydrilla was first reported in Lake Austin in 1999. At that time, coverage was low enough to qualify for a Tier I response which entailed an immediate response toward eradicating the problem within a small area (Chilton 2018). An official task force created in 1999 to discuss, evaluate, and develop SAV control measures comprised numerous governmental entities including City of Austin (COA) Watershed Protection, Parks and Recreation, and Water and Wastewater Departments; the Lower Colorado River Authority (LCRA); Travis County; and TPWD Inland Fisheries. The LCRA also formed a Lake Austin Advisory Panel representing local civilian stakeholders that drafted a resolution to support the task force management plan. In 2000, the task force completed an integrated management plan. However, hydrilla expanded in coverage during the devel- opment of this plan. Thus, the response level was reclassified from Tier I to Tier II, which meant the plant could only be managed as a maintenance function (i.e., no eradication) due to the per- ceived difficulty in full eradication at a larger scale. A review of hydrilla treatment options found that biological control was the most viable option, but initial treatment proposals using triploid grass carp were twice denied by TPWD due to concerns about SAV overgrazing. Hydrilla control in Texas has generally been very con- servative since aggressive grass carp stocking to control hydrilla at Lake Conroe in the 1980s led to complete SAV eradication and angler uproar (Bettoli et al. 1993). Instead, TPWD designed a con- servative stocking regime to prevent total eradication of SAV in Lake Austin and funded a radio-tag study during simulated flood conditions to assess potential escapement, which was found to be negligible (Bonner et al. 2002, Chilton and Magnelia 2008). In 2003, two non-government organizations won a temporary restraining order against TPWD that prevented sterile (triploid) grass carp stocking state-wide. These groups agreed to withdraw their motion in exchange for a science-based resolution evaluating statewide stockings approaches. Lake Austin was accepted as a test 2024 JSAFWAcase to explore conservative incremental triploid grass carp stock- ing approaches (Chilton and Magnelia 2008). The goal for Lake Austin was for hydrilla coverage not to exceed 40 ha (COA 2000). This goal was considered a balanced approach by TPWD biologists that would meet the interests of the various stakeholders. The stocking rate of grass carp was generally less than 11 fish ha–1 (or 25 fish ac–1) of hydrilla. However, with the onset of a drought- of-record in 2009, reduced flows through the reservoir and sub- sequent warmer water temperatures are believed to have favored the rapid and substantial expansion of hydrilla to a peak in 2012 (Figure 1). Increased hydrilla biomass in the reservoir led to con- cerns among controlling authorities that a sudden large flow event could dislodge a significant amount of hydrilla biomass that would damage property and infrastructure as had occurred previously on Lake Austin (Gilroy 2005). Further, vociferous anecdotes of hydrilla-related drowning and hazardous boating conditions ex- pressed by citizens in the late 2000s increased pressure for more frequent stockings. Therefore, public safety and protection of in- frastructure, which are primary reservoir functions, took prece- dence when altering the SAV control strategy. Over 39,000 grass carp were stocked from 2011–2013 at a rate of 15–60 fish ha–1 (Figure 1), which rapidly increased abundances, resulting in more than 56,700 triploid grass carp stocked between 2003 and 2013. The subsequent loss of SAV in the reservoir after 2013 suggests grazing rates exceeded SAV reproductive potential (van der Lee et al. 2017). Figure 1. Lake Austin submerged aquatic vegetation (SAV) coverage (% of total reservoir surface area, vertical gray bars, right y-axis) and cumulative triploid grass carp stocked (# stocked, solid circles, left y-axis) between 1997 and 2022. Hydrilla Management and Bass Populations Bellinger and De Jesús 16 Methods Aquatic Vegetation Surveys Routine aquatic vegetation surveys of Lake Austin were con- ducted by TPWD (De Jesús and Farooqi 2017). To estimate areal coverage of SAV (ha) from these boat surveys, vegetation bound- aries were identified with GPS, sight, and sonar and the total area was determined with geographic information system (GIS) map- ping. Plant species composition was determined from random rake samples periodically pulled during each survey (De Jesús and Farooqui 2017). In response to hydrilla establishment, survey fre- quency was increased from once per year in the 1990s to two to three times per year in 2001. Increased monitoring in spring and sometimes summer was designed to closely track the changes in areal hydrilla coverage in response to triploid grass carp estimated abundance after stocking events. For this study, if multiple surveys were conducted in a year, we averaged the coverage observations to derive mean annual SAV coverage. Fish Sampling and Population Metrics Lake Austin fishery surveys were conducted by TPWD between 1997–2022 and included two types: a comprehensive survey, con- ducted in in 1997, 2000–2001, 2004, 2008, 2012, 2016–2017, and 2020, in which largemouth bass, sunfish (Lepomis spp.), and giz- zard shad (Dorosoma cepedianum) were collected, and a large- mouth-bass-only survey, which was conducted in all other years from 2000–2022. Both survey types were completed in October or November at 12–18 sites per survey year, with sites randomly dis- tributed throughout the lake. Boat electrofishing was done at each sample site for 5 min, with catch per unit effort (CPUE) expressed as number of fish caught per hour (fish h–1). Fish were processed alive in the field and released except for largemouth bass kept for aging purposes. A total of 390 discreet sample sites evenly distrib- uted throughout the reservoir were surveyed between 1997–2022. Prey fish were identified, counted, and measured (TL; mm). Sunfishes were composed primarily of redbreast sunfish (L. aura- tus), bluegill (L. macrochirus), and redear sunfish (L. microlophus), and were subsequently grouped at the genus level for all analy- ses. Largemouth bass were measured (TL, mm) and weighed (g). Sagittal otoliths were collected during comprehensive survey years and in 2017. Prior to 2008 largemouth bass of varying sizes were aged; however, after 2008, only 13 largemouth bass 330–405 mm were randomly selected per year for aging. The change was made to determine the approximate time needed to reach the statewide harvestable minimum length of 356 mm. 2024 JSAFWAData Analyses Largemouth bass proportional stock density (PSD) was calcu- lated using the standard-length categories found in Neumann et al. (2012). Body condition was assessed using relative weight (Wr ; Neumann et al. 2012). Largemouth bass CPUE and Wr were calcu- lated for all fish stock size and larger (CPUE-203 and Wr-203), and for those larger than the statewide minimum-length limit (CPUE- 356 and Wr-356). A Wr of 100 has been suggested to reflect con- dition of good habitat and abundant prey (Murphy et al. 1991), but Wr values as low as 85 have been deemed acceptable in Tex- as reservoirs (M. De Jesús, TPWD, personal observation). If the lake-wide mean Wr of largemouth bass is below 85, there may be deficiencies in the prey population (e.g., small sizes, low biomass), and/or strong density dependent interactions negatively impact- ing largemouth bass condition (Schindler et al. 1997, Fox and Neal 2011). The proportion of gizzard shad small enough to be suscep- tible to largemouth bass predation (<200 mm) was expressed as an index of vulnerability (IOV; DiCenzo et al. 1996), although the net mesh size (~1.27 cm [~0.5”] bar mesh) potentially selected for larger gizzard shad. Sample years were divided into two periods to examine changes in fish metrics due to SAV. Periods were defined as SAV presence (1997–2013) and absence (2014–2022). To test for the negative im- pacts of reservoir aging on fishery quality (Miranda et al. 2010), we first applied the Mann-Kendall test (M-K S; α = 0.05) to determine whether there was a monotonic decline in fishery metrics through time. Differences in mean CPUE, PSD, Wr, and age at 330–405 mm length between SAV periods were examined using a Student’s t-test (α = 0.05). A Mann-Whitney (M-W) U test statistic was used to test for a significant change in largemouth bass age reaching har- vest size between periods of SAV presence and absence. Gizzard shad IOV and largemouth bass age data were log10-transformed prior to analysis to normalize the data. Analyses were conducted using the Real Statistics Resource Pack (Zaiontz 2023) and Sigma- Plot (Systat Software, Inc. 2018). Hydrilla Management and Bass Populations Bellinger and De Jesús 17 Results Submerged Aquatic Vegetation Average SAV coverage from 1997–2013 was 150.1 ± 24.7 ha (mean ± 95% CI). This translated to approximately 22% of the res- ervoir area, varying from 3% (2008) to 40% (2012; Figure 1). De- clines in SAV appeared coincident with triploid grass carp stock- ing (Figure 1). Since 2013, annual SAV total coverage has been less than 0.5 ha system-wide; however, in 2022 SAV patches of south- ern naiad (Najas guadalupensis), Eurasian watermilfoil, water cel- ery (Vallisneria americana), and pondweeds (Potomogeton spp.) were observed re-establishing throughout the reservoir. Largemouth Bass A total of 2581 largemouth bass were collected across all survey years, with 1885 (73%) >203 mm TL, of which 640 were greater than 356 mm TL. System-wide average TL during the SAV period was 325 ± 9 mm, and after 2013 was 331 ± 12 mm. Largemouth bass PSD after 2013 increased 8% (Table 1; Figure 2A). However, largemouth bass population size structure did not significantly dif- fer either through time (M-K S = –17, P = 0.65) or between SAV periods (t22 = –1.2, P = 0.25; Fig. 2A). Largemouth bass stock catch rates (CPUE-203) across years varied from 36 fish h–1 to 114 fish h–1 whereas harvest size catch rates (CPUE-356) ranged from 7 fish h–1 to 38 fish h–1 (Table 1; Figure 2B). Only the CPUE-356 significantly declined through time (M-K S = –43, P < 0.05). Average CPUE-203 and CPUE-356 during the period of SAV presence was 69.4 ± 11.5 and 23.2 ± 5.6 fish h–1, respectively. After 2013, rates declined to 44.0 ± 11.6 and 16.3 ± 4.5 fish h–1 for CPUE-203 mm and CPUE-356 mm large- mouth bass, respectively. Only CPUE-203 significantly declined af- ter 2013 (t22 = 2.66, P = 0.01), although CPUE-356 trended down- ward after 2013 (t22 = 1.55, P = 0.14; Table 1; Figure 2B). Condition of largemouth bass declined over time for both stock and harvest-sized fish (M-K S < –107, P < 0.05; Figure 2C ). Mean Wr-203 peaked at 99 in 2001 and declined to 78 in 2018. Mean Wr- 356 peaked at 104 in 2001 and declined to 79 in 2022 (Figure 2C ). Table 1. Ranges of metrics for Lake Austin, Texas, largemouth bass and prey fish for 1997–2022 and mean ± 95% CI for the periods of submerged aquatic vegetation (SAV) presence (1997–2013) and absence (2014–2022). Metrics include catch per unit effort (CPUE; fish h–1), proportional stock distribution (PSD), relative weight (Wr ), and total length (TL; mm). Asterisks denote significant t-test comparisons of fish metrics between periods of SAV and No SAV: *P < 0.05, **P < 0.001. Largemouth Bass Gizzard Shad Sunfish Period All years SAV No SAV CPUE-203 CPUE-356 PSD 36.7–114.0 65.8 ± 10.3* 44.0 ± 11.6 7.8–37.5 21.9 ± 4.8 16.3 ± 4.5 39.1–78.4 53.3 ± 4.5 58.4 ± 8.2 Wr -203 78.5–98.7 Wr -356 79.3–104.6 CPUE TL CPUE TL 21.5–109.3 221.3–337.4 132.7–480.0 91.7–128.1 92.0 ± 1.8** 97.9 ± 2.2** 56.9 ± 26.1 267.7 ± 34.1 275.2 ± 95.5 107.8 ± 10.0 83.1 ± 1.7 85.6 ± 2.0 63.8 ± 45.8 268.8 ± 8.2 247.6 ± 58.0 99.9 ± 11.8 2024 JSAFWAHydrilla Management and Bass Populations Bellinger and De Jesús 18 Prey Species Comprehensive surveys collected more than 750 gizzard shad, with CPUE ranging from 21 fish h–1 to 109 fish h–1 (Figure 3A; Ta- ble 1). Most gizzard shad (~80%) were greater than 200 mm TL (i.e., IOV <20%; Figure 3B). Gizzard shad CPUE was similar through time (M-K S = 4, P = 0.74) but TL increased over time (M-K S = 36, P < 0.01; Figure 3). Gizzard shad mean CPUE and mean TL were similar between SAV Periods (t7 = –0.28 to –0.04, P > 0.79; Table 1). Over 3200 sunfishes were collected, with CPUE ranging 130– 480 fish h–1 (Figure 3C). Most (67%) sunfishes collected were less than 125 mm TL (Figure 3D). Mean CPUE and mean TL were similar between SAV periods (t7 = 0.38 to 0.97, P > 0.36; Table 1), but both increased over time (M-K S 26–36, P < 0.01; Figure 3). Discussion With the loss of SAV from Lake Austin in 2013, stock-sized largemouth bass electrofishing catch rates and body condition sig- nificantly declined, corroborating anecdotal claims from anglers. Prior to 2013, CPUE-203 was comparable to or greater than other U.S. lakes and reservoirs (Hansen et al. 2015, Schoenebeck et al. 2015). Fluctuations in fishery metrics observed prior to 2013 likely reflected inter-annual differences in SAV extent (5–35%). For ex- ample, the decline in CPUE-203 between 2008 and 2010 may have reflected changes in fish catchability or natural population varia- tions following SAV coverage declines from 2007 to 2009 (Bayley and Austen 2002, Brown and Maceina 2002). However, as SAV coverage increased after 2010, there was a corresponding increase in largemouth bass CPUE. Changes and general improvements in fishery quality with deliberate management, but not wide-spread elimination, of hydrilla coverage have been documented in nu- merous reservoirs across the southeastern U.S. (Wrenn et al. 1996, Brown and Maceina 2002, Sammons et al. 2005). The largemouth bass PSD across all years (50–80) is suggestive of a fishery managed for trophy fish (Willis et al. 1993). Addition- ally, prior to 2013 more fish showed Wr >85, especially for fish >356 mm TL, suggesting favorable habitat conditions for foraging and growth (Maceina and Murphy 1988, Blackwell et al. 2000). Productive habitat is one of several supporting factors needed to sustain a trophy fishery at Lake Austin, along with Florida bass genetics, selection for growth, reduced mortality from high angler release rates, and prolonged growing seasons. These factors likely combined to create trophy fish production that was manifested by a large increase in legacy-class (>5.9 kg) ShareLunker fish caught by anglers during this period of desired SAV coverage. Of the 21 legacy-sized largemouth bass caught in Lake Austin and donat- ed to the program since 1994, 18 were caught while hydrilla was present. Lake Austin was nationally ranked in the top 10 bass lakes Figure 2. Temporal patterns of A) largemouth bass proportional stock density (PSD; mean ± 95% CI); B) catch per unit effort (CPUE; fish h–1) for total length (TL) >203 mm (closed circles) and TL >356 mm (open circles); and, C) relative weight (Wr ) for TL >203 mm (closed circles) and TL >356 mm (open circles) (mean and 95% CI) collected during electrofishing surveys. Annual average submerged aquatic vegetation (SAV) coverage (gray bars; % total reservoir area; right y-axis) also shown. Between periods, mean Wr-203 and Wr-356 were lower after SAV loss (t22 = 6.48 to 7.79, P < 0.001; Table 1). System-wide, over 70% of largemouth bass had Wr > 85, but that proportion declined by almost half after 2013. The average age of a largemouth bass be- tween 330–405 mm prior to 2013 was 2.6 ± 0.2 years (n = 67), but following the loss of SAV, the average age for this group increased to 3.5 ± 0.6 years (n = 26; M-W U = 542, P = 0.002). 2024 JSAFWAHydrilla Management and Bass Populations Bellinger and De Jesús 19 Figure 3. Temporal patterns of catch per unit effort (CPUE; fish h–1) and mean ± 95% confidence error total length (TL; mm) for gizzard shad (A & B; left y-axis) and sunfish (C & D; right y-axis), respectively. Annual average submerged aquatic vegetation (SAV) coverage (gray bars; % total reservoir area; middle y-axes) also shown. in the nation for two consecutive years by Bassmaster Magazine. Although anglers still report trophy-size catches (i.e., 3.6–5.5 kg) from Lake Austin, no legacy-size fish were reported caught again in the reservoir until 2022. In the short-term, losing areas to target concentrated populations of largemouth bass as habitat becomes scarcer makes catching quality fish more difficult (Sammons et al. 2003). However, long-term habitat depletion, caused by extended herbivory pressure, would be expected to hinder trophy fish pro- duction potential. Although negative impacts to largemouth bass condition were evident after 2014, our results suggest that Wr also declined be- tween 2011 and 2013, when hydrilla coverage was greatest (30– 40%; De Jesus and Farooqi 2017). Similarly, excessive growth of hydrilla in the Spring Creek arm of Lake Seminole negatively affected largemouth bass Wr relative to other sections where hy- drilla extent was lower (Brown and Maceina 2002). The tempo- rary Wr decline in Lake Austin could have been due to the dense topped-out hydrilla stands impeding largemouth bass foraging success (Colle and Shireman 1980, Savino and Stein 1982). Thus, there is likely an upper limit of SAV extent that maximizes benefits to largemouth bass. Above these limits, SAV coverage should be controlled for best largemouth bass performance. The largemouth bass condition declines throughout the study likely reflecting the well-documented negative effects of reservoir aging and residential development on largemouth bass popula- tions (Miranda et al. 2010, Gaeta et al. 2011, Miranda and Krog- man 2015). The sudden loss of SAV (and especially hydrilla) after 2013 appears to have amplified the natural aging effects. The PSD 2024 JSAFWAof nearly 60 coupled with the apparent declines in CPUE of small- er largemouth bass after 2013 could indicate recruitment issues to adult bass due to the loss of hydrilla (Bettoli et al. 1993, Webb et al. 1994). Growth of young largemouth bass to harvest sized adults also appeared to decline as noted by the increase in age of bass >356 mm TL. The influence of SAV loss did not appear to negatively affect the forage fish captured in Lake Austin, as gizzard shad and sunfish population CPUE and lengths did not significantly decline after the loss of SAV. In fact, TL of both groups and sunfish CPUE ap- peared to increase through time. In contrast, the sunfish popula- tion in Lake Conroe, Texas, collapsed after the loss of hydrilla due to grass carp stocking (Bettoli et al. 1993). However, the infrequen- cy of sampling may be obfuscating actual population changes and patterns. Survey results did suggest the Lake Austin sunfish pop- ulation overall is dominated by small fish (<150 mm TL), which may indicate resource limitations (Garvey et al. 2002, Aday et al. 2005). Small sunfish can be less optimal prey items for adult large- mouth bass (Blackwell et al. 2000). Additionally, few gizzard shad that were caught would be susceptible to largemouth bass preda- tion based on the observed IOV (DiCenzo et al. 1996). Threadfin shad (D. petenense) also are common in Lake Austin and an im- portant prey option for largemouth bass. However, few threadfin shad and small gizzard shad were netted during surveys, contrib- uting a possible bias in reported IOV values, as well as inhibiting our ability to quantify any changes in these potentially significant prey resources. Management Implications This study has documented that concomitant with the loss of SAV, largemouth bass densities and body condition can be nega- tively impacted. Prior to 2013, a robust trophy fishery was main- tained alongside the active SAV management strategy sustaining a plant assemblage within a desired range of 10–30% SAV coverage, similar to recommendations for other southern U.S. reservoirs (Brown and Maceina 2002, Gilroy 2005, Bonvechio and Bonve- chio 2006, Chilton and Magnelia 2008). A trophy largemouth bass fishery is a desired final management goal for many southern U.S. reservoirs, and SAV plays a critical supporting role in meeting this outcome. While primary reservoir operations will often constrain management efforts, controlling authorities should always consid- er the multiuse aspect of reservoir systems, including economical- ly important fisheries (Miranda 1996). This study also highlights though the long-term difficulties managing an aggressive non-native aquatic plant in the face of stochastic, uncontrollable environmental conditions and compet- ing municipal and recreational reservoir uses. Interannual density Hydrilla Management and Bass Populations Bellinger and De Jesús 20 and coverage of hydrilla are sensitive to reservoir flow rates (Sam- mons et al. 2005, Shivers et al. 2018) which dramatically declined through Lake Austin during the drought. Conditions favorable for rapid hydrilla expansion could become more frequent, as climate projections suggest increased frequency and duration of drought conditions (Nielsen-Gammon et al. 2020). Large-scale harvesting or herbicide treatments are not feasible in Lake Austin because of its use for municipal drinking water, thus future hydrilla con- trol will likely involve triploid grass carp stockings. Future man- agement of habitat and fisheries in Lake Austin should learn and adapt from past strategy implementation and system responses. For example, future SAV extent determinations and control ef- forts should incorporate volumetric assessments to supplement areal coverage when determining the effects of stocked triploid grass carp densities to better inform decisions about supplemental stockings of these fish. Diminishing vertical densities (biomass) of hydrilla observed during surveys in 2012 suggested grass carp had started to widely reduce SAV biomass which should have discour- aged additional stocking. Unfortunately, only the areal coverage of hydrilla was used during the planning of continued stocking, leading to overstocking. Until recently, the abundance of grass carp combined with a return to normal hydrologic management in 2015 continued to suppress SAV growth outside of herbivore exclusion pens meant to serve as founder colonies for plant re-establishment (Bellinger and Schad 2019). However, abundant patches of SAV, comprised primarily of Eurasian watermilfoil and southern naiad (Najas guadalupensis), were finally observed in 2022. This is presumably due to triploid grass carp densities declining low enough to allow plants to begin regrowing. Beyond responsible SAV management to prevent another decade of SAV absence, riparian trees, shore- line vegetation, and CWH should be preserved and enhanced to the greatest extent possible to improve ecological conditions that will also bolster the largemouth bass fishery. Structure and habitat could be preserved or enhanced with incentives, development reg- ulations, active habitat restoration, and installation of littoral hab- itat and living shorelines to help slow the natural reservoir aging process. Finally, history dictates that a collaborative management approach amongst stakeholders is crucial toward understanding and balancing the needs and desires of all interest groups (Chilton et al. 2008). Acknowledgments We thank the numerous reviewers at the WPD, TPWD, and anonymous referees that provided feedback and edits on this manuscript. 2024 JSAFWALiterature Cited Aday, D. D., D. E. Shoup, J. A. Neviackas, J. L. Kline, and D. H. Wahl. 2005. Prey community responses to bluegill and gizzard shad foraging: Im- plications for growth of juvenile largemouth bass. Transactions of the American Fisheries Society 134:1091–1102. Bayley, P. B. and D. J. Austen. 2002. Capture efficiency of a boat electrofisher. Transactions of the American Fisheries Society 131:435–451. Bellinger, B. J. and A. Schad. 2019. Summary of aquatic vegetation restoration efforts in Lake Austin and Lady Bird Lake (2004–2018). SR-19-11. City of Austin Watershed Protection Department, Austin, Texas. <http:// www.austintexas.gov/watershed_protection/publications/document .cfm?id=332112>. Accessed 1 December 2023. Bettoli, P. W., M. J. Maceina, R. L. Noble, and R. K. Betsill. 1992. Piscivory in largemouth bass as a function of aquatic vegetation abundance. North American Journal of Fisheries Management 12:509–516. _____, _____, _____, and _____. 1993. Response of a reservoir fish commu- nity to aquatic vegetation removal. North American Journal of Fisheries Management 13:110–124. Blackwell, B. G., M. L. Brown, and D. W. Willis. 2000. Relative weight (Wr ) status and current use in fisheries assessment and management. Reviews in Fisheries Science 8:1–44. Bonner, T., T. Arsuffi, C. Thomas, and B. Littrell. 2002. Preliminary report: movement and emigration rate of radio-tagged grass carp in Lake Austin, Texas. Report of Texas State University to the City of Austin, Texas. Bonvechio, K. I. and T. F. Bonvechio. 2006. Relationship between habitat and sport fish populations over a 20-year period at West Lake Tohopekaliga, Florida. North American Journal of Fisheries Management 26:124–133. Brown, S. J. and M. J. Maceina. 2002. The influence of disparate levels of sub- mersed aquatic vegetation on largemouth bass population characteristics in a Georgia reservoir. Journal of Aquatic Plant Management 40:28–35. Carpenter, S. R. and D. M. Lodge. 1986. Effects of submersed macrophytes on ecosystem processes. Aquatic Botany 26(C):341–370. Chen, R. J., K. M. Hunt, and R. B. Ditton. 2004. Estimating the economic im- pacts of a trophy largemouth bass fishery: Issues and applications. North American Journal of Fisheries Management 23:835–844. Chilton, E. W. 2018. Aquatic vegetation management in Texas: A guidance document. Texas Parks and Wildlife Department TPWD PWD PL T3200- 1066, Austin. _____ and S. J. Magnelia. 2008. Use of an incremental triploid grass carp stocking strategy for maintaining vegetation coverage in a riverine Tex- as reservoir. Pages 543–555 in M. S. Allen, S. M. Sammons, and M. J. Maceina, editors. Balancing fisheries management and water uses for impounded river systems. American Fisheries Society, Symposium 62, Bethesda, Maryland. _____, M. A. Webb, and R. A. Ott. 2008. Hydrilla management in Lake Con- roe, Texas: A case history. Pages 247–257 in M. S. Allen, S. M. Sammons, and M. J. Maceina, editors. Balancing fisheries management and water uses for impounded river systems. American Fisheries Society, Sympo- sium 62, Bethesda, Maryland. City of Austin (COA). 2000. Lake Austin hydrilla management plan. Wa- ter Quality Report Series COA-ERM 2000-1, Austin, Texas. <http:// www.austintexas.gov/watershed_protection/publications/document .cfm?id=186283>. Accessed 1 December 2023. Colle, D. E. and J. V. Shireman. 1980. Coefficients of condition for largemouth bass, bluegill, and redear sunfish, in hydrilla-infested lakes. Transactions of the American Fisheries Society 109:521–531. _____, _____, W. T. Haller, J. C. Joyce, and D. E. Canfield. 1987. Influence of hydrilla on harvestable sport-fish populations, angler use, and angler ex- penditures at Orange Lake, Florida. North American Journal of Fisheries Management 7:410–417. Hydrilla Management and Bass Populations Bellinger and De Jesús 21 De Jesús, M. J. and M. Farooqi. 2017. 2016 fisheries management survey re- port, Austin Reservoir. Inland Fisheries Division Monitoring and Man- agement Program, Texas Parks and Wildlife Department, Federal Aid in Sport Fish Restoration, Performance Report, Project F-221-M-2, San Marcos. DiCenzo, V. J., M. I. Maceina, and M. R. Stimpert. 1996. Relations between reservoir trophic state and gizzard shad population characteristics in Alabama reservoirs. North American Journal of Fisheries Management 16:888–895. Fox, C. N. and J. W. Neal. 2011. Development of a crowded largemouth bass population in a tropical reservoir. Proceedings of the Annual Conference of the Southeastern Association of Fish and Wildlife Agencies 65:98–104. Gaeta, J. W., M. J. Guarascio, G. G. Sass, and S. R. Carpenter. 2011. Lakeshore residential development and growth of largemouth bass (Micropterus salmoides): A cross-lakes comparison. Ecology of Freshwater Fish 20:92– 101. Garvey, J. E., R. A. Stein, R. A. Wright, and M. T. Bremigan. 2002. Exploring ecological mechanisms underlying largemouth bass recruitment along environmental gradients. Pages 7–23 in D. P. and M. S. Ridgway, editors. Black bass: Ecology, conservation, and management. American Fisheries Society, Symposium 31, Bethesda, Maryland. Gilroy, M. 2005. Hydrilla (Hydrilla verticillata) growth on Lake Austin, Austin, Texas 2005. SR-06-03. City of Austin Watershed Protection Department. <http://www.austintexas.gov/watershed_protection/publications/docu ment.cfm?id=186295>. Accessed 1 December 2023. Hansen, J. F., G. G. Sass, J. W. Gaeta, G. A. Hansen, D. A. Isermann, J. Lyons, and J. M. Vander Zanden. 2015. Largemouth bass management in Wis- consin: intraspecific and interspecific implications of abundance increas- es. Pages 193–206 in M. D. Tringali, J. M. Long, T. W. Birdsong, and M. S. Allen, editors. Black bass diversity: Multidisciplinary science for conser- vation. American Fisheries Society, Symposium 82, Bethesda, Maryland. Maceina, M. J. 1996. Largemouth bass abundance and aquatic vegetation in Florida lakes: An alternative interpretation. Journal of Aquatic Plant Management 34:43–47. _____ and B. R. Murphy. 1988. Variation in the weight-to-length relationship among Florida and Northern largemouth bass and their intraspecific F1 hybrid. Transactions of the American Fisheries Society 117:232–237. Miranda, L. E. 1996. Development of reservoir fisheries management para- digms in the twentieth century. Pages 3–11 in L. E. Miranda and D. R. DeVries, editors. Multidimensional approaches to reservoir fisheries man- agement. American Fisheries Society, Symposium 16, Bethesda, Mary- land. _____ and R. M. Krogman. 2015. Functional age as an indicator of reservoir senescence. Fisheries 40:170–176. _____, M. Spickard, T. Dunn, K. M. Webb, J. N. Aycock, and K. Hunt. 2010. Fish habitat degradation in U.S. reservoirs. Fisheries 35:175–184. Murphy, B. R., D. W. Willis, and T. A. Springer. 1991. The relative weight in- dex in fisheries management: status and needs. Fisheries 16(2):30–38. Neumann, R. M., C. S. Guy, and D. W. Willis. 2012. Length, weight, and as- sociated indices. Pages 637–676 in A. V. Zale, D. L. Parrish, and T. M. Sutton, editors. Fisheries techniques, 3rd edition. American Fisheries So- ciety, Bethesda, Maryland. Nielsen-Gammon, J. W., J. L. Banner, B. I. Cook, D. M. Tremaine, C. I. Wong, R. E. Mace, H. Gao, Z.-L. Yang, M. F. Gonzalez, R. Hoffpauir, T. Gooch, and K. Kloesel. 2020. Unprecedented drought challenges for Texas water resources in a changing climate: What do researchers and stakeholders need to know? Earth’s Future 8:e2020EF001552. Nohner, J. K., W. W. Taylor, D. B. Hayes, and B. M. Roth. 2018. Influence of aquatic macrophytes on age-0 largemouth bass growth and diets. Trans- actions of the American Fisheries Society 147:758–769. 2024 JSAFWAPípalová, I. 2006. A review of grass carp use for aquatic weed control and its impact on water bodies. Journal of Aquatic Plant Management 44:1–12. Roth, B. M., I. C. Kaplan, G. G. Sass, P. T. Johnson, A. E. Marburg, A. C. Yannarell, T. D. Havlicek, T. V. Willis, M. G. Turner, and S. R. Carpenter. 2007. Linking terrestrial and aquatic ecosystems: the role of woody habi- tat in lake food webs. Ecological Modelling 203:439–452. Sammons, S. M., M. J. Maceina, and D. G. Partridge. 2003. Changes in behav- ior, movement, and home ranges of largemouth bass following large-scale hydrilla removal in Lake Seminole, Georgia. Journal of Aquatic Plant Management 41:31–38. _____, _____, and _____. 2005. Population characteristics of largemouth bass associated with changes in abundance of submersed aquatic vegetation in Lake Seminole, Georgia. Journal of Aquatic Plant Management 43:9–16. Savino, J. and R. Stein. 1982. Predator-prey interaction between Largemouth Bass and Bluegills as influenced by simulated, submersed vegetation. Transactions of the American Fisheries Society 111:255–266. Schindler, D. E., S. I. Geib, and M. R. Williams. 2000. Patterns of fish growth along a residential development gradient in north temperate lakes. Eco- systems 3:229–237. _____, J. R. Hodgson, and J. F. Kitchell. 1997. Density-dependent chang- es in individual foraging specialization of Largemouth Bass. Oecologia 110:592–600. Schoenebeck, C. W., S. A. Lundgren, and K. D. Koupal. 2015. Using elec- trofishing catch rates to estimate the density of largemouth bass. North American Journal of Fisheries Management 35:331–336. Shivers, S. D., S. W. Golladay, M. N. Waters, S. B. Wilde, and A. P. Covich. 2018. Rivers to reservoirs: hydrological drivers control reservoir func- tion by affecting the abundance of submerged and floating macrophytes. Hydrobiologia 815:21–35. Hydrilla Management and Bass Populations Bellinger and De Jesús 22 Slipke, J. W., M. J. Maceina, and J. M. Grizzle. 1998. Analysis of the recreation- al fishery and angler attitudes toward hydrilla in Lake Seminole, a South- eastern reservoir. Journal of Aquatic Plant Management 36:101–107. Smith, C. S. and J. W. Barko. 1990. Ecology of Eurasian Watermilfoil. Journal of Aquatic Plant Management 28:55–64. Smith, S. M. and D. J. Orth. 1990. Distribution of largemouth bass in relation to submerged aquatic vegetation in Float Top Lake, West Virginia. Pro- ceedings of the Annual Conference of the Southeastern Association of Fish and Wildlife Agencies. 44:36–44. Systat Software, Inc. 2018. SigmaPlot 14. San Jose, California. van der Lee, A. S., T. B. Johnson, and M. A. Koops. 2017. Bioenergetics mod- elling of grass carp: Estimated individual consumption and popula- tion impacts in Great Lakes wetlands. Journal of Great Lakes Research 43:308–318. Webb, D. H., L. N. Mangum, A. L. Bates, and H. D. Murphy. 1994. Aquatic vegetation in Guntersville reservoir following grass carp stocking. Pages 199–209 in Proceedings of the Grass Carp Symposium. U.S. Army Corps of Engineers, Vicksburg, Mississippi. Willis, D. W., B. R. Murphy, and C. S. Guy. 1993. Stock density indices: De- velopment, use, and limitations. Reviews in Fisheries Science 1:203–222. Wrenn, W. B., D. R. Lowery, M. J. Maceina, and W. C. Reeves. 1996. Rela- tionships between largemouth bass and aquatic plant abundance in Guntersville Reservoir, Alabama. Pages 382–393 in L. E. Miranda and D. R. DeVries, editors. Multidimensional approaches to reservoir fisher- ies management. American Fisheries Society, Symposium 16, Bethesda, Maryland. Zaiontz, C. 2023. Real Statistics Using Excel. <https://www.real-statistics.com/>. Accessed 1 December 2023. 2024 JSAFWA